(source: Electronics World, Dec. 1963)
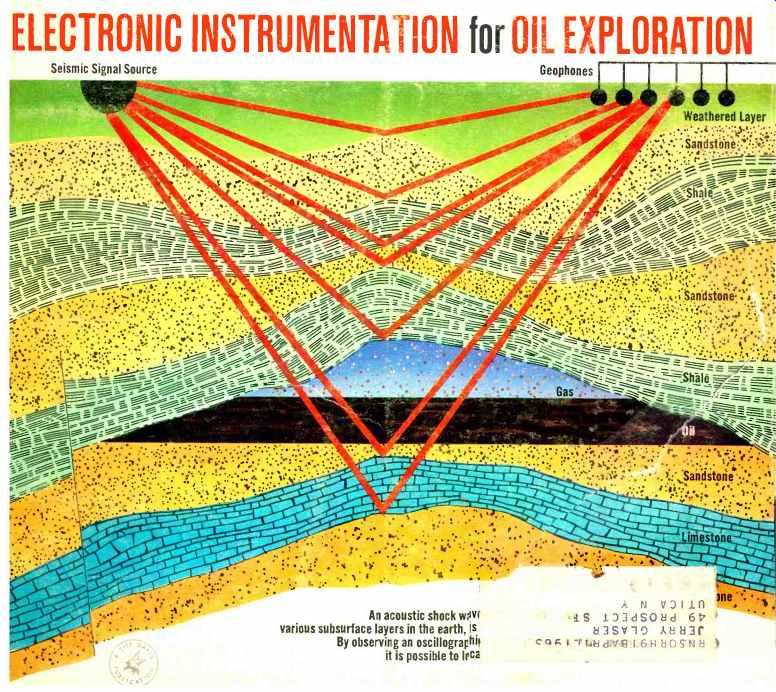
OUR COVER illustrates the subsurface layers in a cross-section of earth
that is typical of the structure found with an oil deposit. Note how the
oil is trapped in the anticline structure, which is an up-fold, bend or
arch in the rock strata.
Acoustic shock waves are reflected from the various sub-surfaces, are
picked up by a number of microphone -- like transducers (geo-phones),
and their signals are passed through electronic circuits to a recording
oscillograph or special tape recorder. For details on this important
technique for finding oil, see our lead story below (Illustration by
Otto E. Markevics.) ...
By LOUIS E. FRENZEL, Jr. / McCollum Laboratories, Inc.
Geophysical prospecting using electronics has led to many oil
discoveries. Acoustic sounding is now done with transistorized equipment,
oscillographs, and computers.
ALL of the major fields of science and technology have profited greatly
by the use of electronic techniques over the past years. Persons in the
fields of chemistry, physics, biology, medicine, and engineering are beginning
to realize the great potentials that electronic techniques offer. Electronic
instrumentation, when used, improves existing conditions in addition to
offering new avenues of approach unheard of before the use of electronics.
One field that has "discovered" electronics is geophysics.
Geophysics is the science and study of the earth and its various phenomena.
Years ago, this was the only definition of geophysics, but today, because
of advances in sciences like electronics, geophysics is now the study
of our solar system and space as well as the earth. Geophysics includes
the fields of geology, geodesy, seismology,, techno-physics, volcanology,
oceanography, petrology, geochemistry, geochronology, meteorology, hydrology,
aeronomy, geomagnetism, solar physics, and others. These are called the
geosciences.
One of the most interesting fields is seismology, particularly the seismic
oil exploration branch of this field. Oil is one of our most important
natural resources and the need for large quantities of oil continues to
exist. Oil and its byproducts have many important uses in our world today,
and new uses are continually being found. For these reasons it is important
that we be on the lookout for new sources of this valuable product. The
major oil companies of this country conduct a constant search for new
petroleum deposits in the earth. Geophysical prospecting techniques using
electronics developed by these and other companies have led to many successful
discoveries of oil deposits.
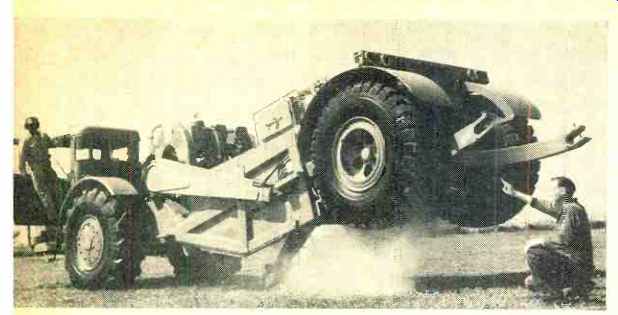
Fig. 1. A gas-explosion chamber built into this 18-ton vehicle is used
to produce an acoustic shock wave for oil exploration.
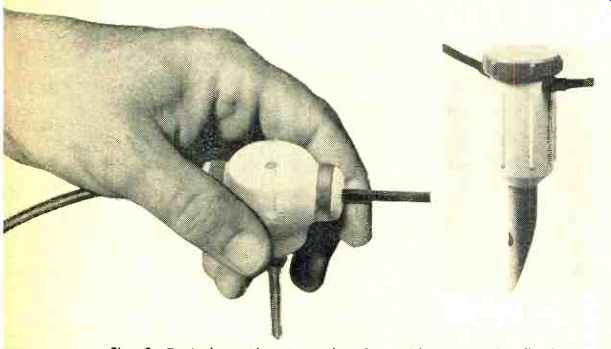
Fig. 2. Typical geophones employed to pick up sound reflections.
Reflection Seismology
Geophysical or seismic prospecting is the science of searching for petroleum,
gas, or mineral deposits by making physical measurements on the earth.
While many different techniques have been devised for making these measurements,
one of the most effective and widely used in oil exploration is reflection
seismology. In this technique, sound waves are generated in the earth.
These waves travel through the earth and are reflected back to the surface
from the various subsurface layers. These reflections are recorded, and
the depth of the layers are computed by knowing the reflection times and
the velocity of propagation. From this data, special maps of the subsurface
are plotted. These maps are then interpreted by geophysicists who are
able to determine if the subsurface structures are those which are usually
oil bearing in nature.
If results of these measurements show conditions favorable to oil accumulation,
further tests will be made and a pilot well may be drilled.
Reflection seismology is an acoustic-sounding technique.
In this technique, a generator source is used to produce a sound or shock
wave that will penetrate the earth and be reflected back to the surface.
The most widely used method of generating seismic waves is by detonating
a charge of dynamite or other explosive. A hole, called a shot hole, is
bored into the earth and the explosive is placed in it. The explosive
is then detonated and a large impulse shock wave is produced. The wave
travels through the earth in all directions and is reflected from the
various subsurface layers. Reflections as deep as 20,000 feet have been
recorded.
A newer method, developed by Burton McCollum, involves the dropping of
a weight. A three-ton metal weight mounted on a large truck is dropped
from a height of approximately nine feet. This creates a tremendous shock
wave in the ground. This technique produces reflections which are just
as useful as those produced by a dynamite blast.
Electric and hydraulic vibratory sources which vibrate the earth in a
sinusoidal manner have also been used as seismic signal sources, but many
of these are still largely experimental. The "Vibroseis ", a
vibratory exploration technique developed by the Continental Oil Company,
is already being used commercially.
(Editor's Note: Still another method of producing the shock wave was
announced recently by Sinclair Oil Corp. A gas explosion chamber is mounted
in the center of an 18-ton large-wheeled diesel-driven vehicle, called "Dinoseis." The
chamber is held against the ground surface by part of the weight of the
truck. When the gas mixture is exploded, a 100,000 foot-pound seismic
impulse is produced (Fig. 1).) After the acoustic energy is radiated,
it will travel through the earth with a velocity which is dependent upon
the media through which it passes. Basic physics says that the velocity
of propagation of acoustic energy is dependent upon the type of material
through which the energy passes and other factors. For example, the velocity
of sound waves is higher in rock than it is in clay soil. The earth's
subsurface is made up of many different types of layers of rocks, soil,
sand, and other substances. The velocity of propagation will change abruptly
as the acoustic wave passes from one layer to another. Because of this
velocity change, a reflection is produced. The exact nature of the reflection
will depend whether the signal goes from a high-velocity zone to a low-velocity
zone or vice versa. This reflection takes the form of a very small vibration
of the earth which travels upward vertically toward the surface. This
reflection is actually a low-frequency (10 to 300 cps ) sound wave.
Detection and Recording
The next step in this oil exploration technique is the detecting and
recording of the vertical reflections. Here is where electronics plays
a major role. Fig. 4 shows a cross section of the earth and a block diagram
of the equipment used in the reflection method.
The reflections are picked up by a microphone-like transducer called
a geophone. Fig. 2 shows two typical geophones.
The geophone, also called a seismometer or detector, is usually buried
several inches in the earth. Care is taken to see that the geophone makes
good contact with the earth so that it moves when the earth moves during
a reflection.
A typical geophone consists of a coil of wire which is suspended by a
spring and which moves in the field of a permanent magnet. When a reflection
appears, a small vibration in the earth will be transmitted to the geophone
and will cause motion between the coil and the permanent magnet. This
causes a voltage to be induced into the coil which is a function of the
nature of the vibration. The geophone is made so that it will respond
best to vertical movement. A cable attached to the geophone carries the
voltage to other equipment.
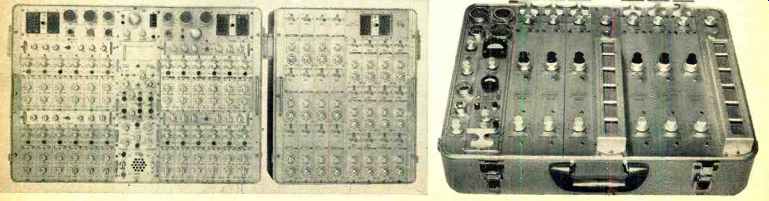
Fig. 3. Typical low-noise, high-gain, multichannel transistorized geophysical
amplifiers and filter units.
A single geophone is never used in practical work. A large number of
geophones are arranged in various patterns over a large area to obtain
diversity. Diversity improves signal-to-noise ratio. The geophones are
wired together in various series-parallel combinations in order to obtain
a satisfactory impedance match to other equipment.
The reflection signals from the earth are extremely weak.
Even though a large amount of energy is radiated by, say, a charge of
dynamite, the voltage produced by a group of geophones is still too small
to record directly. Noise in various forms also complicates natters. Just
as in communications and radar systems, the signal-to-noise ratio is important
and steps must be taken to improve it; otherwise, good naps of the subsurface
will not be obtained.
The output voltage from the geophones is fed to a low noise, high-gain
amplifier. This low-frequency amplifier provides extremely high gains
in the 10- to 300-cps range. Care is taken in the design of these amplifiers
to ensure a low noise figure. Fig. 3 shows two such geophysical amplifiers.
These amplifiers contain built-in low-and high-pass filters which help
to improve signal-to-noise ratio by eliminating unwanted frequencies above
and below the reflection signal frequency. These filters are normally
of the constant-K LC type and are made variable to provide the desired
filtering.
Some amplifiers also contain a 60-cps notch filter to eliminate power-line
interference which tends to be a problem in many areas.
All good geophysical amplifiers contain a.g.c. circuits. In many cases
even a form of delayed-a.g.c. is used. This a.g.c. system improves performance
by allowing the amplifier to operate over a wide range of input signal
amplitudes without distortion. Another feature of some geophysical amplifiers
is programmed gain. Programmed-gain circuits allow the gain of the .amplifier
to change with time. Since it takes a longer time for deep reflections
to return to the surface than it does shallow reflections, naturally the
deeper reflections will show up much smaller in amplitude on the record.
In some cases it may take five or six seconds from the time the seismic
signal was radiated for the most greatly attenuated, deeper reflections
to return to the surface. The amplifier with programmed gain changes its
gain with time and thereby brings all the reflection signals to approximately
the same amplitude level.
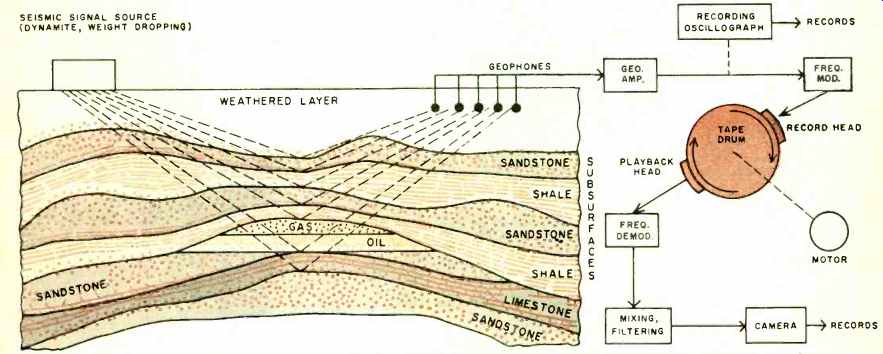
Fig. 4. Cross section of earth showing the subsurface layers and a block
diagram of associated electronic instrumentation.
After considerable amplification and some filtering, the signals are
ready to be recorded. In order for the geologist or geophysicist to analyze
the reflection information, it must be recorded and put into a suitable
form. The most common form is a photographic record showing a number of
reflection recordings. These are normally arranged so that the reflection
signals from each dynamite blast or weight drop appear one beneath the
other. In this way, line-ups of reflection signals will be easier to detect.
This record is made by applying the seismic signal to a light galvanometer.
The light is flashed onto light-sensitive paper which is moved past the
galvanometer. This paper is processed and a record is produced. Timing
lines are also put onto the record so that the geophysicists may know
how long it takes the radiated signal to travel into the ground and to
be reflected back to the surface. These timing lines are usually graduated
in steps of 10 milliseconds, and the entire record can be anywhere from
four to six seconds long. A device for producing such a record is called
a recording oscillograph, and a typical unit is shown in Fig. 5. Fig.
5 also shows a seismic reflection record made on a similar recording oscillograph.
Magnetic tape recording systems are also used, particularly in poor record
country where additional filtering and other processing must be done to
obtain usable reflection data.
The magnetic tape record can be sent to a central laboratory for processing.
A record is made by placing a magnetic tape about four inches wide and
three feet long on a large rotating chum. The magnetic record and playback
heads are placed adjacent to this drum. The drum revolves as dynamite
shots or weight drops are produced and the resulting reflection signals
are recorded on the tape.
The recording technique actually involves frequency modulation. The amplified
reflection signals frequency-modulate a high audio-frequency carrier (2-5
kc.) . It is this modulated carrier that is recorded on the tape. This
FM technique results in a better recording than would be obtained if no
modulating system were used. It also eliminates the need for a bias signal
which is usually required in magnetic tape recording systems to reduce
distortion inherent in magnetic recording. Although FM is generally favored,
standard magnetic tape biasing techniques are also used successfully in
recording systems of this type.
A typical FM modulator consists of a free-running multi vibrator which
oscillates at a frequency of 4 kc. The frequency of this oscillator is
dependent upon the RC time constant used in the grid circuits. This oscillator
is frequency modulated by the reflection signals. A tube acting as a variable
resistance is connected in the grid circuit. As the incoming reflection
signals are applied to this tube, its resistance changes, thus changing
the circuit time constant and the frequency of the oscillator.
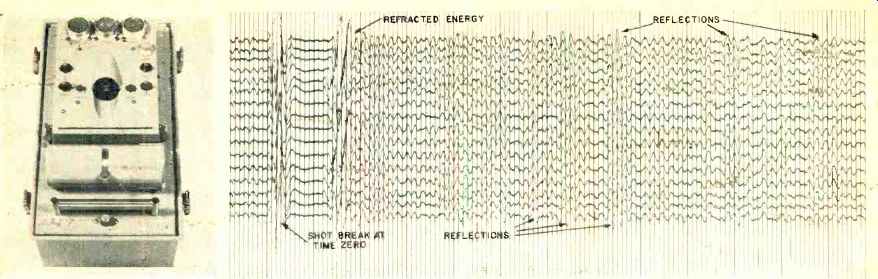
Fig. 5. (Left) A recording oscillograph of the type that is employed
to produce seismic records for use in oil exploration. (Right) Typical
seismic reflection record. Artificially induced shot break indicates when
seismic energy was first radiated. Refracted energy is the first arrival
of seismic signal at the geophones. This is energy refracted from the
first high-velocity zone below the surface. The reflections indicate a
change in velocity or the boundaries of reflecting layers. Reflections
are picked by noting where the signals of each trace line up or where
the peaks and troughs appear to fit into one another. Other reflections
besides the ones that are obvious in this figure can be picked by close
observation.
The FM signal from the playback head is amplified and clipped and made
into a varying-frequency rectangular wave.
It is then differentiated and the resulting spikes trigger a monostable
multivibrator. One fixed-amplitude, constant duration pulse is produced
by the monostable circuit for each trigger spike. The pulses are then
integrated or averaged in a suitable low-pass filter. The output is the
original geophysical signal.
After the reflection signals have been recorded, they can be processed
in various ways. Mixing (compositing) and filtering are the most commonly
used processes, and both methods improve the signal-to-noise ratio.
After a number of shots have been recorded, the resulting reflection
signals can be mixed together. The reflection signals may be obscured
by noise in all of the recordings, but because' the noise tends to be
random it will cancel to some extent. The reflection signals, however,
being the same on each record, will add. Practice has shown that the signal–to-noise
ratio improves approximately as the square root of the number of records
composited. Each trace in Fig. 5, for example, is actually a composite
of 320 individual records.
In addition to the filtering in the geophysical amplifiers, special filters
are used in cases where the signal-to-noise ratio is low. These filters
have many configurations and are largely of the analog type. Digital-filtering
techniques have also been developed. Because of the transient nature of
seismic signals, filter requirements are rigid and care must be taken
to see that the filter does not distort the signals and give false results.
In addition to filtering and mixing, processing also includes corrections.
In order for the geophysicist to obtain a true picture of the various
subsurface layers, there are certain characteristics of the earth that
must be taken into consideration.
The uppermost layer of the earth, the weathered layer, will give false
reflection data if it is not corrected. Since it varies in thickness with
location, information regarding this weathered layer must be obtained
before suitable reflection data can be obtained.
Ground elevation and geophone placement must also be considered. Since-the
geophones are spread over a large area, the elevation may be different
for some geophones than for others. Also the distance between the signal
radiator and the geophones may be changed during the shooting. Connections
must be made. Other corrections of various kinds must also be made depending
on the equipment and methods used.
Obtaining the Information
To obtain information about the oil-bearing properties of a particular
part of the earth, a crew of men is sent into the field along with the
equipment just described. Since all of the work is done in the field,
the equipment must be portable.
It is made so by mounting it in trucks. For dynamite operations, shot-hole
drilling apparatus must be taken, and with the weight-drop technique,
a large truck must be provided to carry the weight and its associated
equipment.
An instrument or recording truck carries most of the electronics. The
recording equipment and amplifiers are mounted in this truck. Because
of the complexity of this truck, a skilled electronics field technician
must be present to set up, operate, and maintain this unit in the field.
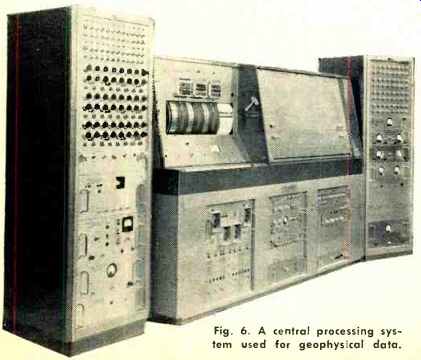
Fig. 6. A central processing system used for geophysical data.
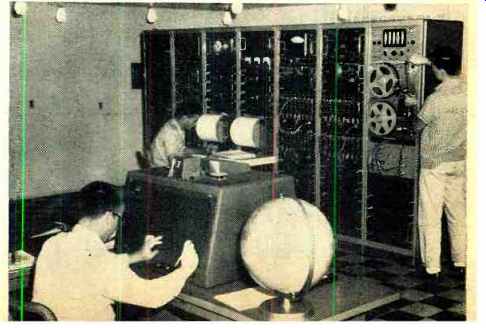
Fig. 7. One of our nuclear explosion detection stations.
The equipment used in oil exploration is sometimes mounted in boats so
that water areas may be worked. Many oil discoveries have been made beneath
large bodies of water--such as the Gulf of Mexico.
While most of the work of producing data for the geophysicist is done
in the field, many times recordings are sent to a central processing point.
The processing center may do all or part of the filtering, mixing, and
correcting usually necessary and, in addition, may handle the work of
several field crews. A typical central processing unit is shown in Fig.
6.
Other Exploration Techniques
The exploration technique described here
is only one of a number of methods used in finding oil and investigating
the interior of the earth. Others include refraction seismology, magnetic
and gravity measurements, and well logging. Like reflection seismology,
most of them are dependent upon electrical or electronic instrumentation.
Refraction seismology is quite similar to the reflection method in that
it requires almost exactly the same equipment. The technique makes use
of the refracting properties of the subsurface layers. This technique
is generally inferior to the reflection method because it does not give
an exact picture of the subsurface. It is useful in many instances, however,
particularly where it is desired to determine the velocity of propagation
in certain areas.
In well logging, a deep hole is drilled in the earth and instruments
are lowered to the bottom. As the instruments are pulled up, measurements
are made on the rock and soil and a chart or log is plotted as a function
of depth. Among the properties measured are acoustic velocity, electrical
resistivity, density, self potential, gamma-ray radiation, and others.
Geologists have said that this is one of the most useful of all of the
various exploratory techniques used.
The Mohole and Vela Uniform
While a large percentage of the electronic instrumentation used in the
geosciences is concerned with locating oil and gas deposits, electronics
is also helpful in other areas. Two recent interesting uses of electronics
in the geosciences are the drilling of the Mohole and Vela Uniform.
The Mohole project is an attempt to drill through the outer crust of
the earth into the mantle or inner earth. The mantle comprises about 80%
of the earth's volume, but little is known of its character. Knowing the
nature of the inner earth will aid scientists in proving or disproving
theories and the history of the earth.
The Mohole will be drilled at sea because the mantle is closer to the
surface under the sea than it is under land.
Naturally, this brings on many complications. The drilling ship must,
at all times, be accurately positioned over the hole being drilled regardless
of the condition of the weather and the sea. This requires a special drilling
ship as well as an excellent positioning system. The positioning system
will make extensive use of electronic techniques. Electronic instruments
will also be used to log the hole as it is drilled. Reflection and refraction
seismic tests have been run over the water areas that are being considered
as drilling sites. We can expect to see some interesting results of the
experiment.
Vela Uniform is part of the government's research project for the development
of a satisfactory arms control in the form of an underground nuclear blast
detection, identification, and location system. The entire program is
dependent upon electronic instrumentation. Seismological observatories
or detection stations will be set up at carefully chosen locations over
the world. These stations will be equipped with seismometers, amplifiers,
processing and recording instruments, and other devices necessary to the
detection of nuclear blasts and other seismic disturbances.
Underwater seismometers with telemetry equipment will also be used. Fig.
7 shows a typical detection station for nuclear explosions.
As advances are made in electronics, so will advances be made in the
geosciences. It is hard to predict what lies ahead but it will no doubt
be of considerable value to mankind-and much of it because of the contributions
made by electronics.
|