For many years, audible differences among phono preamplifiers have been
noticed by careful listeners.
With the introduction of transistorized preamplifiers, these differences
became more obvious, leading to the widely-held impression that the audible
quality of preamplifiers is related to the basic technology employed. Phonograph
preamplifiers have been ranked using conventional tests of frequency response,
noise, distortion, etc., however, subjective judgments of "quality" will
yield different results. Frequently subjective judgments have sent engineers
back to their test benches to ascertain what aspect of performance caused
specific listener reactions, and in many cases new tests were instituted
to characterize the differences. A case in point is that of crossover distortion
in early transistor power amplifiers; engineers by and large did not consider
measurements of low-output level distortion important until listener reactions
prodded them to do so.
Ultimately, the object of all measurement must be considered to be the assessment
of the subjective quality of the device under test. Since conventional tests
are widely considered as inadequate to characterize quality, new tests which
represent real-world conditions more accurately are necessary. The reasons
for the existence of new tests are that the usual test signals employed do
not adequately represent the demands of program material, and the results
of tests should be weighted for human perception or annoyance value. One
weighting which is frequently employed is to equalize noise measurements
for the well-known fact that the human hearing mechanism is not particularly
sensitive to low and very high frequencies at low levels as first described
by Fletcher-Munson. Such weighting helps to correlate objective measurement
with subjective assessment. Yet, in many other areas, weighting ought to
be considered necessary, but adequate standards to correlate with perception
have not yet been developed. For example, total harmonic distortion is just
that, total. The regular test gives equal weight to all harmonics, whereas
it is clear that, due to the masking effect of a tone on its close-in harmonics,
ninth harmonic distortion should be given far more weight than second.
There are three basic forms of testing, and they may be assigned an order
based on ease of replication of the results. The first is electrical tests
conducted by engineers with a collection of equipment which measure various
aspects of performance. This form of testing is the easiest to replicate
and communicate since the hardware produces numbers. Methodology may vary
from laboratory to laboratory, so that some small differences may be expected,
but for identical, well-specified measurements, high correlation is usually
found. Of course, such measurements form the basis of published specifications
and test reports.
The second form of testing is the audible A-B comparison of a device under
test versus a known standard device. If certain precautions are observed
(such as matching levels carefully), results of such tests usually correlate
well from listener to listener.
While the convenient handle of numbers is much harder to hang on these results
than with electrical tests, complex statistical analysis of paired comparisons
(such as brighter-duller on a scale from 1 to 5) may be used to produce numerically
significant results which may be correlated.
The third form of testing is the extended listening test. While the least
easy to replicate, this form has been responsible for discovery of performance
areas like crossover distortion.
Since this form is the most like the environment in which we listen everyday,
it may be said to be occurring continuously. If many people in different
settings with different program material come to identical impressions about
a piece of equipment without prejudice from market forces, then, more than
likely, that area of performance is one which, while not characterized by
conventional tests, is nevertheless real. In a number of cases in recent
history, awareness of performance properties has grown from a conviction
held by many based on lengthy listening to engineering tests with numerical
confirmation.
When we came to investigate phonograph preamplifier performance, it quickly
became clear that there were audible differences among designs that had nearly
identical measurements on conventional tests.' The most obvious difference
to observers on a level-matched, instantaneously switched, A-B comparison
was that the perceived frequency response did not correlate with standard
measurements.
Cartridge-Input Impedance Interactions
Typical cartridges of the moving magnet or variable reluctance variety consist
of a coil of wire wound on a core. Such an arrangement may be mostly characterized
electrically by a resistance in series with an inductance. The inductive
property implies that the impedance of the cartridge rises dramatically with
ascending frequency. Therefore, the loading provided by the input impedance
of the phonograph preamplifier in parallel with the cable capacitance also
becomes increasingly important with ascending frequency. For example, at
1 kHz, a typical cartridge has an impedance of about 1.5 kilohm, but at 20
kHz, its impedance may be about 50 kilohm. Since one standard input impedance
is 47 kilohm, the input impedance and cable capacitance form a voltage divider
with the source impedance, rolling off the electrical high frequency response
by about 6 dB. Cartridge designers make use of this fact of life by tailoring
the mechanical system of the cartridge in an inverse manner to the electrical
response to yield a substantially flat overall response. However, since the
source impedance approaches the load impedance at the highest audible frequencies,
the cartridge becomes increasingly sensitive to the load at higher frequencies.
Cartridge designers absolve themselves of responsibility by stating the proper
load for their cartridges e.g. "load impedance 47 kilohm, 300 pF." Unfortunately,
many tonearm/cable makers do not specify capacitance, and virtually no preamplifier
maker specifies input impedance completely.
The degree of this problem is by no means trivial. It is not unusual to
find a variation of ± 3 dB from desired response due to this effect. A recent
survey of phonograph preamplifier input impedances used the criteria that
the input impedance of the preamplifiers should be able to be modeled by
a 47 kilohm ±10% input resistance in parallel with a capacitance of from
0 to 200 pF. Only 11 units of 26 tested met this criteria. An additional
three units of the 26 could generally be characterized as having input resistance
and capacitance in the right range, but could not be completely modeled by
an equivalent R and C. Twelve other units had more serious interaction, including
one with over 600 pF input capacitance and a number with input resistance
as low as 35 kilohm. Also--there was no good correlation within a given manufacturer's
line or with price.
In a system with unknown interaction, two techniques may be employed to
ameliorate the interaction problem. One is to use a cartridge with fairly
low inductance, as it will be less sensitive to loading than high inductance
cartridges (low 200 to 300 mH, high 600 to 800 mH). Alternatively a buffer
amplifier may be built and inserted between the phonograph cartridge and
the preamplifier input. The buffer amplifier terminates the cartridge in
47 kilohm in parallel with a chosen input capacitance and may serve as a
sonic reference for comparison with preamplifiers with unknown input impedance.
There is a small penalty paid in noise for such an arrangement, however,
proper frequency response usually subjectively outweighs a small noise contribution.
The schematic for such a buffer amplifier is shown in Fig. 1
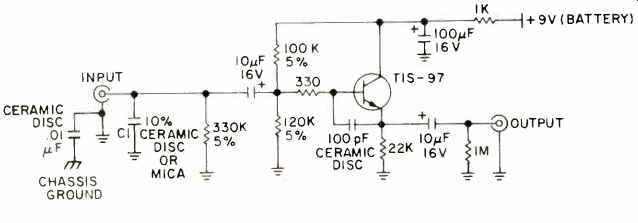
Fig. 1--Preamplifier input buffer. See text for choice of C1. Only one channel
is shown.
If you have a system in which you know the rated load impedance of the cartridge,
the cable capacitance of all interconnecting phono cables, and the input
resistance and capacitance of your preamp, you can determine the proper value
for a cartridge termination capacitance and add that value across the input
of your preamplifier.
An easy way to do this is to use a short Y adapter cable (the Switchcraft
version contributes 20 pF to the total), and solder the proper capacitor
between the center lug and shell of a phono connector. Connect the cable
from the cartridge to the Y along with the termination capacitor and connect
the Y adapter to the preamp.
There may be several sources for the cartridge/input interaction. An important
source are capacitors used for eliminating radio-frequency interference directly
across the input to the preamplifier or strapped from base/grid/gate (for
bipolar transistor/tube/field effect transistor inputs respectively) to the
emitter/cathode/ source or from collector/plate/drain to base/grid/gate.
While these capacitors may reduce the susceptibility to r.f.i. each use must
be examined for its contribution to any frequency response error in the audio
band. Amplifier stages also have input capacitance which is dependent on
the devices used and on the topology of the circuit. 2 Another source is
the result of "looking into" an active amplifier at its input terminals.
The open-loop (without the application of feedback) input impedance of a
bipolar transistor is only moderately high (about 50 kilohms); negative feedback
is used to raise the input impedance to a very high value, then an input
termination resistor of, typically, 47 kilohms is wired across the input
terminals to properly load the cartridge. Unfortunately, the amount of available
feedback decreases at high audio frequencies due to stability considerations,
and thus the input impedance falls off with increasing frequency and causes
an interaction.
Descriptive terminology used by listeners to describe frequency response
errors due to cartridge/input impedance interactions runs the gamut from
grittiness, graininess, shrillness, dullness, transistor sound, forward,
recessed, etc. When preamplifiers which demonstrate impedance interaction
are used with a buffer amplifier or are modified so as not to interact, the
differences previously noted from the standard on an A-B test tend to disappear.
A-B testing has demonstrated that in normally operating equipment, overall
level is the most critical parameter to match, followed by frequency response.
Matching the levels and frequency response with a cartridge source usually
eliminates most of the differences between preamplifiers. Since interaction
is a prevalent problem, the assessment of phonograph cartridges is complicated
by the fact that cartridges interact differently with the various input impedances.
Conclusions drawn about cartridges when used with preamplifiers of unknown
input characteristics may be invalid except for describing a particular combination
of cartridge and preamplifier.
Since most differences between phono preamps disappear when the level and
frequency response are matched, the sum of frequency response errors including
RIAA equalization error and cartridge/input error should currently be considered
to be the most important in assessing differences between various pieces
of equipment.
Other Performance Areas
The phonograph preamplifier is a logical place to alleviate another system
problem prevalent today. Many listeners choose fairly high compliance cartridges
and combine them with conventional tone arms. Such a system often has a resonance
between the stylus compliance and the tone arm effective mass plus cartridge
mass in the 7 Hz region. Since few records are really flat, warp frequency
components will lie in the same band as the resonance and will be accentuated.
These warps may not be directly audible by themselves, but they are likely
to cause intermodulation or overload of power amplifiers and loudspeakers.
In a typical system playing at 90 dB SPL three feet from the loudspeaker,
nearly the full woofer excursion is used up in reproducing the warp. This
causes audible intermodulation with the program material. In one case a tape
machine was returned to its manufacturer for a gross form of distortion which
would happen periodically, even accompanied by complete cutoff of the signal.
When nothing wrong could be found with the machine itself, and it was noticed
that the signal cutoff happened with a period equal to one revolution of
a record, the owner was questioned about his record playing equipment. With
the best advice available to him, he had chosen a very highly compliant cartridge
for his massive tonearm. Such a system has a resonance down around 4 Hz which
is in the area of highest-amplitude warp frequencies.' Here the combination
of cartridge mass, very extended infrasonic response in the phonograph preamplifier,
and record warp had conspired to overload the tape recorder with 4 Hz range
garbage.
What was happening to his loudspeakers is interesting to contemplate.
An optimum design for an infrasonic filter is one which greatly attenuates
the region of record warps and tonearm resonances without audible consequences
in the low bass range due to the phase effects associated with such a filter.
A three-pole (ultimate slope 18 dB/octave) filter was studied for its effect.
Such a filter may be designed so that it has no attenuation at 25 Hz, 1 dB
at 20 Hz, a 3 dB point of 15 1/2 Hz, and is 21 dB down at 7 Hz, and 35 dB
down at 4 Hz.
This is adequate attenuation for warps, as may be seen by observation of
woofer cones while playing warped records. To study the phase effects, first
program material was used to ascertain any consequences. When no change in
the character of the bass reproduction was found, a worst case test was conceived,
and an all-pass filter was constructed with the phase response of the infrasonic
filter, but with a flat frequency response. A shaped pulse from a test generator
was passed through the all-pass filter to a power amplifier and headphones
rated flat to 10 Hz. The filter was switched in and out to determine if any
change could be heard. With a training period, very careful listeners barely
perceived the difference. The amount of group delay (the time difference
caused by the phase effects between the extreme bass and the mid-range) introduced
by the filter is 20 mS at 20 Hz. Broadcast and standards organizations have
perceptible group delay standards, since long telephone lines are subject
to phase effects. The German Post Office and Broadcasting Organization has
made 70 mS at 50 Hz the acceptable limit, and the CCIF has made 80 mS at
50 Hz the limit for imperceptibility on program material, while Bell Labs
concludes 70 to 90 mS at low frequencies is inaudible. Since the three-pole
design has better than 10 times less group delay at these frequencies, it
seems probable that such a filter has inaudible phase characteristics.
An interesting filter-related phenomena has been noticed independently by
a number of listeners. On playing somewhat warped records on a level matched
A-B comparison, the unit which contained an infrasonic filter seems to make
the bass sound "tighter." Since this runs contrary to what one
would expect if group delay were a dominant effect, the answer could well
lie with the fact that in the unit which passes the infrasonic warp, the
intermodulation between the warp and the bass colors the program material.
This makes sense if one remembers that the ears' perception of amplitude
and frequency modulation peaks at around 4 Hz, around the same frequency
as the worst warps.
Noise performance of the cartridge/preamp system is also a case of interaction.
The design for noise should account for the fact that normally a cartridge
is connected, rather than a short circuit, as applied in most specifications.
It is possible to design for the short-circuited input test so that the best
numbers are produced; however, such a design will have substantially more
noise with a cartridge connected than will one designed for the real-world
condition with a cartridge. Also, noise design should take into account the
low-level frequency response characteristics of human hearing. When the design
is optimized with a cartridge connected and weighted for human perception,
using good devices, then further improvement is very difficult. Because the
thermal noise associated with the source impedance is the dominant noise
source, and since a commercial pressing rarely approaches the noise level
established by the cartridge and electronics, practical improvements in noise
level are unexpected.
Sine wave input overload has been subjected to a numbers race with limited
meaning. Since the input level is limited by the ability of the cartridge
to track the groove, it is fairly simple to set meaningful criteria. The
worst case combination of high output cartridge and peak recorded velocity
(that cartridge cannot track that record!) yields a number which, when converted
to 1 kHz, is 95 mV rms. It should be emphasized that this is a worst case
condition not likely to be approached in practice. However, the specification
of 1 kHz input overload does not specify the overload characteristics completely
since, in general, the input overload varies with frequency according to
an inverse RIAA function. Since the disc is recorded with the same function,
the overload of the preamplifier is thus fitted to the medium. Any deviation
from such an input overload curve should be noted.
Slew rate is the principal high frequency limitation in most circuits.
Slew rate relates to the ability of the output of the amplifier to move
fast enough to follow every nuance and twist of the input signal completely.
It is expressed in volts/µS referenced to the output of the device under
test.
By various mathematical manipulations, we can change the reference back
to the tip of the stylus and determine the required slew rate at the output
of the preamplifier required to follow the groove. Using specifications for
one of the hottest cutter heads, combined with half-speed cutting, liquid
nitrogen cooling, a high sensitivity cartridge (again, it could not track
this cut), cutting just one pulse, etc., the required slew rate turns out
to be about 0.03 V/µS at the output of the phono preamp. If we understand
that a physical process is involved, actually getting a mechanical stylus
to follow a groove shape, where a mechanical limit is established by the
contact radius of the stylus with the radii of the groove wall, then such
a number does not seem outlandish. And measurements made of "hot" records
yield about one-half this value. Faster slew rates, per se, are unnecessary
to follow the music, however, some margin of safety is useful to prevent
the onset of any nonlinearity. It is thus useful to have a wide power bandwidth
in a preamplifier since any information coming into the input of the preamplifier
above the audio band, whether from the record or from r.f.i., will be detected
as nonlinear behavior, and garbage will be dumped from that process down
into the audio band. Since input r.f.i. filters may cause audible frequency
response errors, the preamplifier may be called upon to handle ultrasonic
signals, with filtering after the preamp. So long as such signals do not
overload the preamplifier in amplitude or in slew rate, they will be passed
cleanly and filtered by a subsequent filter.
Equalized preamplifiers, such as phono preamps, are also subject to a particular
form of intermodulation distortion termed difference-tone intermodulation.
Such distortion arises when two high frequency tones, closely spaced, are
introduced into the device and intermodulate with one another so as to produce
a first order intermodulation product (f2 - f,). Since the equalization boosts
low frequencies almost 40 dB more than the highest frequencies, the inter
modulation product is additionally amplified by the equalization. Inter modulation
of 0.1 percent in a flat amplifier case may be magnified to almost 10% by
equalization. Using measurements from commercial records as a basis for testing,
tones of 13.0 and 13.1 kHz were mixed 1:1 and applied to the input of the
device under test at a composite level of 40 mV rms. Distortion ranged from
1 percent for a simple two transistor topology to less than 0.02 percent
(measurement limit) for the best topologies.
Transient intermodulation distortion relates to an amplifiers' ability to
reproduce a high frequency tone in the presence of transients. A newly proposed
test for transient inter modulation distortion correlates, with some adjustment,
to the square-wave asymmetry test proposed in the JAES article. Otala's test
consists of a 3.18 kHz square wave on which a 15.0-kHz sine wave rides in
a specified level relationship. The combined tone is lowpass filtered by
a 100-kHz filter (6 dB/octave). For the case of an equalized preamp, the
signal is then applied to an inverse-equalizer network, in our case an inverse-RIAA
network, to compensate for the frequency response of the device under test.
The output spectrum of the device under test is then examined for the presence
of intermodulation products which were not present in the input signal.
A test performed using Otala's specified conditions for high-quality equipment
with a composite input level of 85 mV rms yields no measurable inter modulation
products on a topology which produces no measurable asymmetry on the square
wave test described above. Inadequate slew rate could lead to transient intermodulation
distortion. However, the very fast transients which produce this form of
distortion are limited in rise time and level by the finite acceleration
of the stylus and by the electrical low-pass filter formed by the cartridge
source impedance and the load of the cable and input impedance of the preamplifier.
The required rise time and symmetry to pass this test are well beyond what
can be expected from phono cartridges; however, a test which is unusually
sensitive may give an engineer technical information about the source and
form of asymmetries in his circuit.
A new design has developed with input from listening experiments and with
development of test bench procedures designed, in general, to better correlate
to the real-world applications of phono preamps. The object was to meet all
the normal criteria of phono preamps, to pass the new tests as they evolved,
and to do so at the lowest price. The design is detailed in the JAES article,
where a few of its salient features may be seen in the measured performance
of a number of prototype channels. The new tests were passed without sacrifice
of any conventional specifications and with only a small increase in cost
over the simplest units.
References
1. Holman, T., "New Factors in Phonograph Preamplifier Design," J.
Audio Eng. Soc., Vol. 24, pp.
263-270 (May, 1976).
2. J.G. Graeme, G.E. Tobey, and L.P. Huelsman, Operational Amplifiers Design
and Applications, (McGraw-Hill, New York, 1971).
3. L. Happ and F. Karlov, "Record Warps and System Playback Performance," Preprint
926 (D-5), presented at the 46th Convention of the Audio Eng. Soc., New York,
1973.
4. J. Kogen, B. Jakobs, and F. Karlov, "Trackability - 1973," Audio,
Vol. 58, No. 8, p. 16 (Aug., 1973).
5. E. Leinonen, M. Otala, and J. Curl, "Method for Measuring Transient
Intermodulation Distortion," Preprint 1185 (H-6), presented at the 55th
Convention of the Audio Eng. Soc., New York, 1976.
(Source: Audio magazine, Feb. 1977; Tomlinson Holman* [*Chief Electrical
Engineer, Advent Corp., Cambridge, Mass. 02139])
= = = =
|