In the acoustics field, white noise sources are frequently employed along
with octave and one-third octave band filters. Typical applications include
the measurement of transducer characteristics, material absorption-reflection
and transmission coefficients, and room parameters such as reverberation
time. One problem commonly encountered in making these measurements is that
the noise signal detected falls off greatly at low frequencies since the
rms noise passed by the filter varies as the square root of the filter bandwidth,
and hence the center frequency of the filter.
Generally one compensates for this effect lay increasing the power-amplifier
gain when the lower frequencies are to be examined; but, since the filter
is usually connected at the output (Fig. 1), this increased gain can result
in an overload of both the power amplifier and the loudspeaker.
A more practical solution to this problem is to convert the random noise
source from one having a constant energy/cycle, or white noise frequency spectrum,
to a constant energy/octave, or pink noise response. This may be accomplished
by employing a conventional white noise source and connecting a "pink
noise filter" to its output. This type of filter basically has a transmission
characteristic which falls off inversely as the square root of the applied
frequency (Fig. 5). The rms output from such a pink noise source will then
be independent of the octave band being examined (Fig. 2). A simple circuit
for achieving this type of filtering in the audio range from 10 hz to 20 khz
is shown in Fig. 3, with the general form of one of the stages given in Fig.
4.
Since d.c. coupling of the stages is employed, Rb is set equal to zero to
minimize the d.c. gain of each stage, and hence the effect of d.c. offset
voltages on filter stability. Using Fig. 5 as a guide, a-20 dB/decade piecewise
approximation is employed using three stages with their break frequencies
selected to provide the best possible fit to the -10 dB/decade desired
slope. This requires three curves of the type shown in Fig. 4, spaced a decade
apart in frequency, and allows for the use of the same resistors in each stage
by simply changing the capacitor by a power of 10 to shift the breakpoints
to the desired location. The addition of the RC break frequency at f1 = 17.78
kHz at the input of IC3 extends the accuracy of the response to beyond 30
kHz.
It should be noted that when using the filter there is no impedance matching
problem since its frequency characteristics are essentially independent
of the source and load impedances connected to it; in addition, any available
supply voltages from ±6 to ±18 volts can be utilized. If 1 percent resistors
and 5 percent capacitors are employed, the response should be accurate
to within one dB of the desired characteristic.
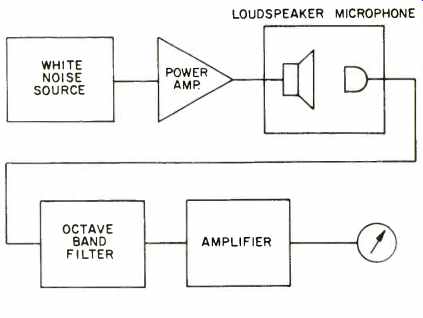
Fig. 1-General measurement technique.
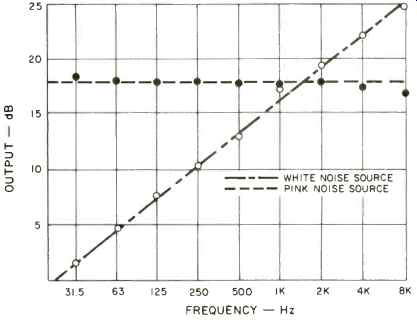 Fig. 2-Actual noise source outputs vs. frequency when examined in octave
bands.
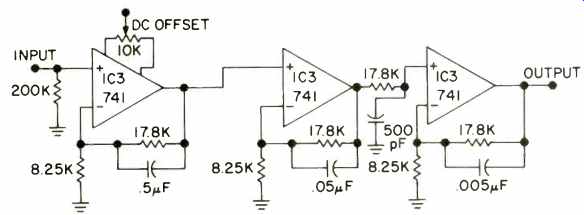 Fig. 3-Audio pink noise filter design.
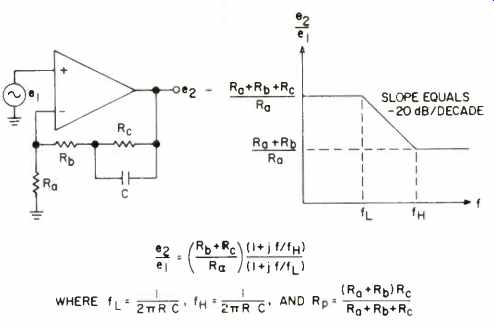 Fig. 4-Single stage characteristic.
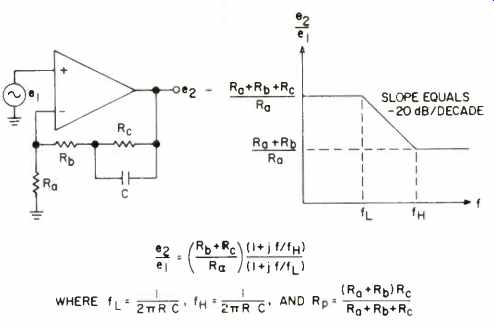
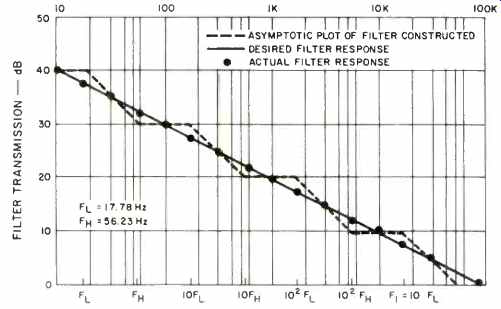 Fig. 5-Actual filter transmission characteristic.
(Source: Audio magazine, March 1977; Dr. Robert Mauro [Assistant
Professor of Electrical Engineering, Manhattan College, Riverdale, N.Y.])
= = = =
|