(source: Electronics World, Aug. 1963)

By WALTER H. BUCHSBAUM, Industrial Consultant
Accuracy goals depend on applications and the duality of instruments being
checked. For any situation. however. satisfactory standards or facilities
can be. worked out.
HOW MUCH precision should one expect and how much can actually be realized
when one is calibrating test instruments? What standards can be or should
be used? How does one go about doing the job or getting it done? The answers
to these questions will depend on who wants the calibration performed, the
type and quality of the equipment whose accuracy is to be checked, and finally
the use to which this equipment will be put. These requirements allow for
a wide variation in the results that will be obtained.
Consider one of the less demanding situations. When a technician wishes
to check the "B +" in a radio or TV receiver, it is not too important
if the nominal 250 volts is actually measured at 262.5 or 237.5 volts.
If voltmeter accuracy is 5 per -cent, this alone could account for the 12.5
-volt difference. Variation in line voltage could just as well be responsible.
If both line voltage and the meter reading are down, only 225 volts might
be read. Deviation would have to be at least this great and probably
larger before the technician would begin to consider the possibility of
a defect. Although the accuracy requirements here are not demanding, they
do exist. If voltmeter error were, say, 10 percent or greater and the user
did not know this, he could be misled.
In industrial and military electronics, on the other hand, standards are
much more critical. Laboratories, factories, and installation facilities
often allocate considerable time and money to the maintenance of test equipment
accuracy. In fact, government contracts conventionally carry clauses requiring
that all test equipment be calibrated carefully against approved standards
at least once every six months.
Reference Standards
In the United States, the exact electrical values for voltage, current,
frequency, resistance, capacitance, and inductance are determined by the
National Bureau of Standards of the Department of Commerce. Laboratory or
secondary standards for these electrical parameters are measured against
the Bureau's primary standards at certain regular intervals and are then
certified as to their accuracy.
Calibration against certified standards is generally a specialized job.
Large organizations maintain special departments for this purpose. Smaller
companies send or bring their equipment to nearby laboratories that offer
a calibration service.
When such service is required on an outside basis, there should be little
difficulty in locating a suitable agency. A request is addressed to the
Bureau of Standards, either at Washington, D.C., or to the office in Boulder,
Colo. The Bureau will advise where standards of the required type are available
in the writer's locality. Each of the three armed services also maintains
standards laboratories, which are available to qualified commercial firms.
A qualified firm is one that holds a contract with the particular service.
It can obtain permission to use standards facilities through the project
director.
All major military bases involving electronics maintain standards facilities.
Well known bases include the Signal Corps at Fort Monmouth, the Naval Research
Laboratories, and the Air Force's Wright Field. For security reasons, the
services avoid publicizing all such locations.
In addition, the Air Force maintains a fleet of trailer trucks, each of
which carries a complete set of calibrating standards. These mobile laboratories
insure precise measurements by making the rounds of various Air Force installations
and enabling frequent checks on test equipment calibration.
Although elaborate facilities of this nature are a far cry from what the
aforementioned technician would need, many of the problems and principles
are common. The technician in a non -critical role is mostly concerned with
calibrating a.c. voltage, d.c. voltage, direct current, and resistance on
his v.o.m. and v.t.v.m. These will be the most important ones, although
there will also be some interest in capacitance, inductance, and frequency.
While the required accuracy is much greater in industrial and military applications,
essentially the same quantities are involved.
There are a number of references and methods that can be used to insure
accuracy in noncritical situations short of comparison against certified
NBS standards. Since these involve measures that may be applicable at any
level of calibration they will be discussed first.
Basic Meter Checks
Conventional voltmeters, used everywhere for measurement of basic electrical
quantities, include the v.o.m. and v.t.v.m. Such instruments are most often
specified to have an accuracy of ±3 per -cent for the d.c. scales, ±5 percent
for the a.c. scales, and still less for resistance measurements. Many users
still overlook the implication of the clearly stated qualification that
the specified accuracy is with respect to full -scale reading.
Consider a typical instrument with a 250 -volt d.c. range and a full-scale
accuracy of +-3 percent. This means it could be off 7.5 volts not only at
250 volts, but at any other reading on that scale. This same voltage discrepancy
would then amount to 7.5 per -cent error if 100 volts is being read. If
15 volts is being measured on the same range, allowable error could be as
much as 50 percent.
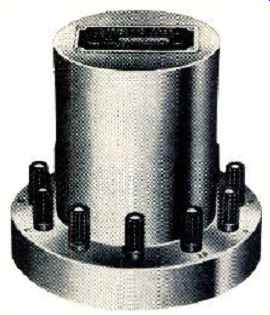
Fig. 1. The Eppley Standard-Cell Voltage Reference, accurate to
.005 ° or better, is tapped in ten steps up to 10.193 volts.
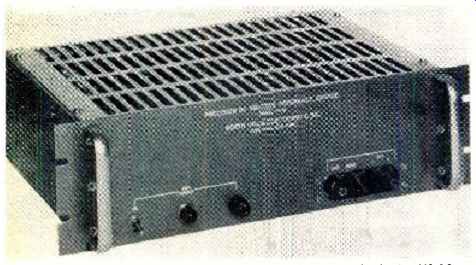 Fig. 2. An a.c.-powered, well-regulated standard, the VS-10 by North Hills
Electronics, provides d.c. reference output.
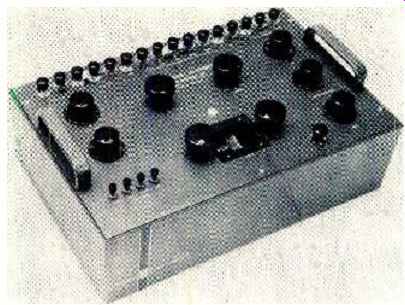
Fig. 3. A universal potentiometer by Leeds & Northup. It combines a
standard voltage reference with precision resistors and a closely calibrated
potentiometer for fine resolution.
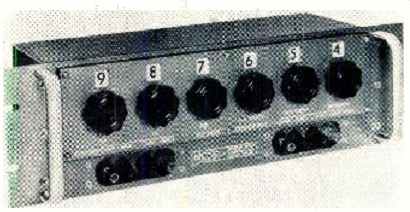
Fig. 4. A resistive voltage divider made by North Hills Electronics. It
is made for use with an external voltage source.
In practice, down-scale accuracy is considerably better than what these
figures suggest. If the meter movement were a perfectly linear device,
percentage accuracy would be the same at any point on the scale. However,
there is some non-linearity in the magnetic meter circuit and some introduced
mechanically by the use of springs and weights that balance the movement.
Since calibration by the manufacturer is made at the full-scale end, the
cumulative effect of errors will be experienced at the low end of the scale.
It is therefore preferable, when possible, to use a range that permits readings
to fall in the higher end of the scale.
The first step in checking a meter is to make sure that zero indication
is accurate. The pointer is set, if necessary, via the external adjusting
screw with the instrument in its normal operating position. The instrument
should be on its lowest d.c. range, with all test leads disconnected.
Meter bearing balance is checked next. While all calibration will be performed
in the instrument's normal operating position, the pointer should show no
tendency to stick when the meter is tilted through any of the three major
axes. If the pointer does not return to zero after tilting, rebalancing
is needed. This is a job for an expert. The instrument manufacturer or an
authorized agency should be used.
If balance is good, d.c. voltage ranges are checked by measuring a known
voltage source. The most elementary source is the widely available 1.5-volt,
carbon-zinc cell which, under certain conditions, will give good accuracy.
To begin with, a large cell with screw terminals is preferred to a flashlight
size. The battery should also be unused, and reasonably fresh. The nominal
voltage usually given for calibration is 1.55 volts. Actually an unused
cell about two years old will probably still measure about that much, whereas
a fresher one may read about 1.58 volts. However, this range of variation
is no more than -±-1%, which is well within the specified accuracy for conventional
meters. As a rule, the owner will consider his instrument does not need
recalibration if it reads anywhere between 1.5 and 1.6 volts. At worst,
error in this case is not likely to exceed ±3 %. Higher d.c. voltages and
scales can be checked by combining single cells in series. This is not recommended,
however, as it builds up margin for uncertainty. Anyone who is really interested
in maintaining a v.o.m. or v.t.v.m., or several such instruments, at good
accuracy should consider investing in something better. Mercury cells, for
example, are available that will maintain accuracy well within 1 percent
over a period of several years. They can be obtained in calibration sets
that include several in a single housing, in a series, with taps between
cells, Still better, so-called "working standard" meters are available
for less than $100. Typical of these is the Weston 931 series, accurate
to ±0.5 %. With one such, a single, accurate voltage source is not necessary.
The instrument to be checked and the working standard monitor any voltage
source or sources simultaneously, and readings at various voltage levels
are compared.
On d.c. voltage checks, the lowest range is always calibrated first. In
some meters, it is possible to adjust the series resistance here if calibration
is seriously off. In most cases, a fixed resistor must be replaced. The
next higher range is checked, and so on up to the highest. Resistors added
in the voltage divider in each successive range are adjusted, if necessary.
The d.c. current ranges are checked out next, starting with the lowest
first If a standard calibration source is not available, a known voltage
and precision resistors are required. Current is calculated with Ohm's Law.
In addition to the value of the calibrating resistors, input resistance
of the meter on each current scale must be considered in calculating current.
Possible error introduced by deviation in the latter resistance value can
be made insignificant by choosing calibrating resistors whose values are
many times higher than that of the meter. Percentage accuracy of the calibrating
resistors is determined by the degree of accuracy required. Proceeding from
current scale to scale, the values of successive meter shunts are corrected
as needed.
Accuracy of the a.c. ranges is affected by frequency and waveshape of the
voltage being measured and by components in the rectifier system, as well
as by divider resistors.
A reasonably good oscilloscope will make a reliable monitor for checking
sinusoidal waveshape and will also indicate voltages in terms of peak-to-peak values, if it incorporates a voltage calibrator or is used in conjunction
with one.
Good waveform is needed because the average meter does not respond to r.m.s.
value directly. It is calibrated to read r.m.s. equivalent value on true
sinusoidal a.c. only. If the scope shows that waveshape of the available
60-cps or 400-cps source is distorted, a good audio oscillator can be used.
To convert scope peak -to -peak readings to r.m.s. equivalents, divide the
former by 2.83 (or multiply by 0.353).
Accurate readings on a.c. may be due to faults in the a.c. rectifier system
in addition to deviations in the divider resistors. Rectifier faults are
most likely to show up as inaccuracy in the lower end of all scales. ( Even
with a good system, meters tend to be less accurate in down-scale readings
than they are on d.c.) Although a calibration resistor is usually included
in the rectifier assembly, the best cure for a fault here is replacement
of the entire system with a new assembly obtained from the meter manufacturer.
Resistance scales are checked last. At best, the ohmmeter function of a
multi -purpose meter is not designed for high precision and should not be
used when such accuracy is required. The ohmmeter tends to be more accurate
near the center of its scale than at either end, so calibration should be
performed with precision resistors whose values fall in the center portion
of each scale. The best one can hope for is good accuracy and scale -to
-scale uniformity in this portion. The degree of error in extreme up -scale
and downscale readings can then be recorded and used to compensate readings
taken subsequently in actual measurement.
More Accurate Standards
Certification of a standard by the NBS does not necessarily mean it is
the most precise type that can be obtained; it simply provides assurance
that the standard does indeed meet whatever accuracy it specifies. How much
certified accuracy should be obtained depends on the instrument being calibrated.
For example, the Eppley Standard -Cell Voltage Reference, shown in Fig.
1, provides ten taps from 1 to 10 volts d.c. with accuracy within ±0.005
%. It is available from the Eppley Laboratory in Newport, R.I., for about
$240. Such cells must be used in bridge circuits where little or no current
is drawn.
An establishment that maintains a considerable number of service -type
voltmeters for ordinary work might consider such an investment. If these
meters are rated at +3% accuracy, the standard would be more than adequate,
as standards should ordinarily be at least twice as accurate as the instruments
they will be used to check. If the establishment also uses a digital voltmeter
or several for more critical work, and the latter is rated at ±0.01 %, purchase
of the Eppley reference would be all the more worthwhile -but the standard
would now be just about sufficient. These cells can be held to ±0.001% if
ambient temperature is controlled.
While the mentioned sources depend on chemical action, there are also voltage
and current references that are purely electronic. One such is the so-called "Solici-cell" in
Fig. 2, available from North Hills Electronics of Glen Cove, N.Y., for $
750. Although a.c.-powered, it depends on a zener diode in a temperature
-controlled box for an output of 10 volts d.c. Its guaranteed accuracy is
also 0.005 %, but it does not suffer from changes due to aging, overload,
and ambient temperature in the way that chemical sources do.
Some instrument companies offer specialized calibration sets that combine
such facilities as voltage and current sources for d.c. and a.c. with precision
resistors, capacitors, and inductors. Weston Instruments of Newark, N.J.
offers such sets. A simple voltage source, after all, is not self -sufficient
for calibrating a variety of ranges.
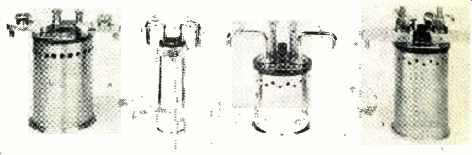 Fig. 5. Thomas standard resistor (left) is rated at 1 ohm to 0.0001 %.
Units to right are other low-resistance standards.
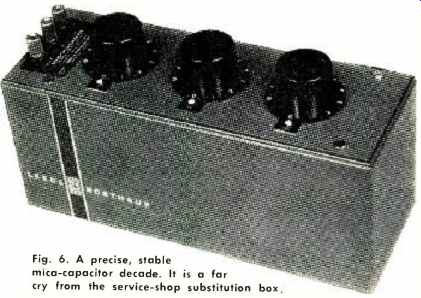
Fig. 6. A precise, stable mica-capacitor decade. It is a far cry from the
service-shop substitution box.
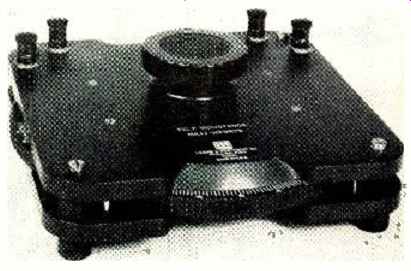
Fig. 7. Three sets of carefully wound coils interact in the Brooks "Inducto-meter" for
inductance readings on a linear scale.
In voltage calibration, it is often advisable to use a standard high enough
for the high scales and employ a precise resistance divider for lower voltage
scales. A "universal potentiometer" of this type, made by Leeds & Northrup
of Philadelphia, Penna., is shown in Fig 3. It combines a standard voltage
source with resistors and a potentiometer of exceptional precision. Voltage
accuracy is rated to ±0.01% and readable output down to 0.1 microvolt is
available. An instrument of this type is also useful in laboratory applications
other than calibration.
A less complex resistance voltage divider is shown in Fig. 4. Designated
model RD-1 by its manufacturer, North Hills Electronics, it is available
for $425. Used in conjunction with an external voltage source, its output
is that fraction of the input which is indicated by the numerals above
each of the decade switches.
For precise resistance calibration, Thomas-type standard resistors (named
after the person who developed them) are available from Leeds or Northrup.
The one shown to the left in Fig. 5 is a 1-ohm unit certified to an accuracy
of 0.0001% if temperature is controlled.
Shown with it are other standard resistors with values of 1 ohm and less.
While d.c. standards and calibration methods have traditionally lent themselves
to greater accuracy than is obtainable for a.c., recent developments have
improved a.c. techniques considerably. Since there is no a.c. equivalent
of the standard cell, most sources of a.c. voltage and current are refined,
electronic signal generators. One company, for example, offers an a.c. standard
at 400 cps that is accurate to ± 0.1% in frequency and = 0.025% in voltage.
Aside from voltage, one dealing with a.c. may want to check such other
parameters as impedance and power factor.
Precise units of inductance and capacitance are called for. Figs. 6 and
7 show devices in this category made by Leeds Northrup. Fig. 6 is a capacitor
box made up of precisely calibrated, highly stable mica capacitors mounted
on high quality decade switches. Its rated accuracy is ±0.1 %, +0.5 pf.
Calibrating capacitors more precise than this set, although they will seldom
be required, are available.
The Brooks "Inductometer" of Fig. 7, available for $550, consists
of three sets of carefully wound coils. One set is mounted in the rotating
disc, which is calibrated in millihenrys. One set of fixed coils is located
above the disc, another below it. When the disc is rotated, mutual-inductance
changes provide the variety of inductance readings possible. The coils have
been carefully designed and shaped so that rotation over 170 degrees produces
an almost linear change. At 1000 cps, the "Inductometer" is accurate
to ± 0.3 %.
Conclusion
The relatively crude procedures outlined for the simple v.o.m. and v.t.v.m.
in the early portion of this article can be improved considerably and also
made easier with some of the devices described subsequently. Also, with
these more refined standards, other types of instruments and parameters
can be checked. Yet, of necessity, the special devices available have only
been touched on. The complex matter of frequency calibration and standards,
for example, has not been treated at all. Actually it would take more than
one volume to give the broad area of calibration and standards comprehensive
treatment.
Nevertheless, much has been achieved if interest in the subject has been
aroused. On the practical level, further information can be obtained from
the National Bureau of Standards and the manufacturers mentioned in this
article on the following: standards, their prices, and organizations that
specialize in providing calibration service.
Another practical alternative is available for individuals or small organizations
that prefer to do their own work but cannot justify the required investment.
If calibration checking is not done on a steady schedule, it is possible
to rent precisely calibrated equipment on a short -term basis, either for
a particular job that requires exceptional accuracy or to check regular
equipment. General Electric's Instrumentation Service in Schenectady, N.Y.,
for example, offers a long list of rental equipment. While the manufacturer's
own line is, of course, fully represented, instruments from other sources
are included on the list.
A Wheatstone bridge by Leeds & Northrup, to cite one example, is available
for $18 a month. G-E also maintains, as do other manufacturers, an instrument
repair and calibration service shop from which trained technicians are available
on an outside-call basis.
Any firm interested in maintaining precision of its measuring instruments
has a wide choice of alternatives that may be fitted in with its needs
and its budgetary considerations.
|