(source: Electronics World, Aug. 1963)
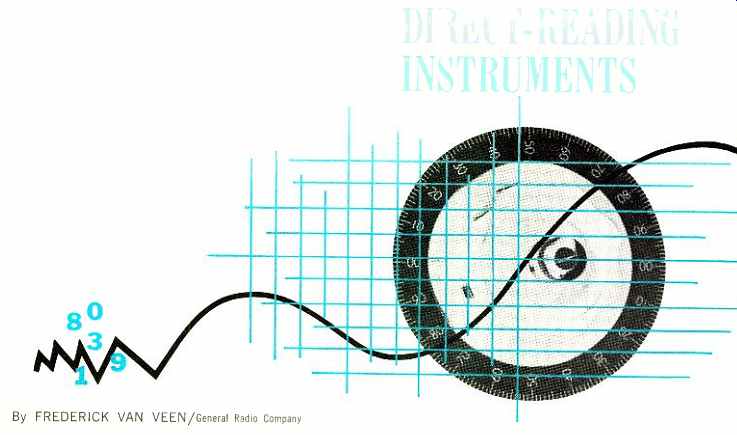
By FREDERICK VAN VEEN /General Radio Company
Significant developments in the past ten years, especially those
that have advanced the art of measuring. New types of test instruments
that measure frequency by counting, record quantities graphically, measure
L, C, R, Z, voltage, and special microwave measuring devices.
THERE has been, over the past decade, a tremendous improvement in electronic
measuring instruments.
The rapid advances in precision, accuracy, and convenience of measurement
have played a vital part in the electronics "boom" that has meant
a 600% rise in sales dollars.
Everything, in a sense, begins with measurements, and the limits of measurement
are the limits of science.
The boom in electronic measuring instruments is a mixed blessing to the
average technician. Ten years ago it was pretty easy to select a bridge
or voltmeter for a specific job. Shopping was a matter of looking at a few
catalogues and inter-comparing some specifications. Then the measurement
industry really expanded, manufacturers outdid one another in offering varieties
of multipurpose instruments, and the catalogues bulged. The 1953 IRE Directory
listed 69 manufacturers of bridges; the 1963 edition lists 140. Under "Graphic
Recorders" the 1953 directory lists 47 companies, the current volume,
145. The 1963 edition lists no fewer than 224 companies making digital counters,
a category not even included in the directory of a decade ago! The hundreds
of different instruments manufactured to measure frequency, impedance, resistance,
inductance, capacitance, voltage, and current represent a wide choice of
accuracies, features, and price. There is also, of course, the duplication
inherent in our competitive system. No one article or series of articles
can do justice to the overwhelming number of direct-reading instruments
available today. The emphasis here will be on the more significant developments
of the past ten years, and especially those that have advanced the state
of the measurement art.
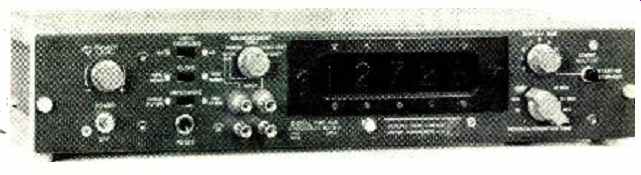
-------- Probably the most important new type of instrument of the past
decade is the frequency meter using digital counting techniques. This
example, the General Radio 1151-A, is a general-purpose counter for laboratory
or production -line use that can measure frequency accurately from a fraction
of a cps up to 300 kc. The unit also measures the period of a cycle and
frequency ratios. Price: $1195.
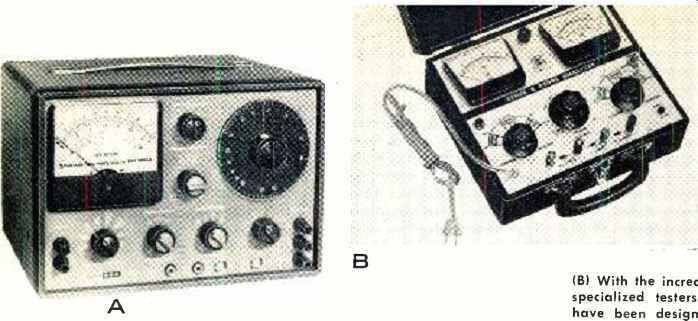 ----------(A) Many direct-reading instruments are highly versatile
since they may combine several functions within a single unit. Our example
is in the audio field. The device shown, the Eico 902, combines an a.c.
v.t.v.m. for accurate audio measurements, and generators and filter circuits
for making both harmonic-distortion and inter modulation-distortion tests.
Such measurements are read directly on the meter face. Distortion figures
are within 5% of full scale and the voltage figures are ±4% of the full-scale
reading. The price is $250, factory-wired.
(B) With the increasing use of semiconductor diodes in equipment have come
specialized testers for checking these components. Many of these testers
have been designed for practical laboratory use as well as for incoming
diode stock inspection. One typical unit, the Seco 210, includes tests
for zener diodes, silicon and germanium power and signal diodes, as well
as selenium rectifiers. Various variable sources of power are incorporated
in the circuit. Results are displayed on two meters. Price of unit: $154.95.
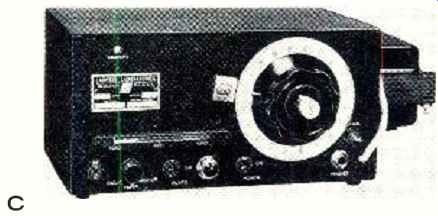 (C) Not all frequency meters are digital devices. Typical of the more common
and, incidentally, less expensive type is the Lampkin 105 -B shown.
This instrument is a heterodyne-type frequency meter with a built-in crystal
calibrator. It is used to measure transmitter frequencies anywhere in the
range of 100 kc. to 175 mc. In addition, it will function as an accurate
weak-signal generator for receiver alignment. Instrument meets FCC requirements
for a frequency monitor in the mobile -radio service below 50 mc.
With some additional equipment, the meter will also meet the FCC accuracy
requirements for the newer split -channel frequencies. The price is $260.
Mechanical and Convenience Improvements
The measuring instruments of 20 or 30 years ago were typically big, black,
square, and heavy. This was simply the way people thought instruments should
look; indestructible, reliable, conservative. But styles change and most
of the black, square instruments went the way of black, square automobiles.
Today's instruments enjoy the benefits of new materials, miniaturization,
and much of what is sometimes called "human engineering" but which
has always been a part of just good mechanical design.
Instrument cabinets now try to be all things to all people; some can be
quickly adapted to relay -rack, bench, or portable use. Then there is the
tiltable cabinet, with a captive cover that serves as an easel -type stand
when the instrument is in use. Extensible front legs on mangy cabinets let
the user look at the front panel head -on whether he is sitting or standing
at the bench. And cabinets of the larger manufacturers come in standard
sizes, so that they can be stacked into a neat arrangement.
Readouts have improved tremendously. The meters are larger with scales
designed for easy reading. And, too, there are now digits -neon digits,
incandescent digits, projected digits, digits in windows, and more digits
on printed tape.
Instruments are lower--the form factor apparently follows that of automobiles.
The day of the three -inch -high instrument has arrived, apparently to
save relay-rack space. Instruments used in the field have become more
portable than ever, as they are transistorized. Also, some instruments with
heavy power demands are starting to "go portable," thanks to the
rechargeable nickel-cadmium battery.
There are so many convenience improvements in instruments that it is difficult
to list them all. But one that deserves special note is the quality of instruction
manuals. Another is the greater availability of manufacturers' sales and
service engineers. Manuals and consulting services can be just as important
as some of the instrument's technical specs.
Instrument Specifications
The buyer of an electronic instrument must be guided by the specifications
published by the manufacturer, but the catalogue shopper who tries to select
an instrument purely by published data had better be on his toes.
Not that the manufacturers aren't truthful. Dishonest specifications are
fortunately a rarity in this business, because they punish their perpetrators
too quickly. But every manufacturer wants to put his best foot forward and
stress the specifications where he is strongest. Also, there are many honest
differences of opinion among manufacturers on just how best to state something.
Take, for instance, the problem of specifying the low limit of measurement
on a v.t.v.m.
Suppose the voltmeter has a low range with a full scale of 10 microvolts,
and an accuracy of ± 10% of full scale. The voltmeter will certainly measure
voltages below 10 microvolts, but a 1microvolt reading might be wrong by
100 %, and most people would not consider that a measurement.
Another aspect of the same problem: a 3% voltmeter is generally more accurate
than a 2% voltmeter if the 3% is 3% of indicated value and the 2% is 2%
of full scale. The moral of all this is that you should not play the numbers
game with instrument specifications, but should read words as well.
One of the best ways to approach laboratory instrument selection is to
consult the manufacturer. Sales engineers who sell such electronic instruments
are, by and large, extremely competent, helpful, and low-pressure. They
will try to steer you to the right choice of instrument, even if it means
steering you to another company. They offer a valuable consulting service
for anyone with a measurement problem, and the wise shopper takes advantage
of it.
But even knowing where to start is a problem. There is a bewilderingly
large number of electronic measuring instruments made by several hundred
different manufacturers to measure more than 200 different electrical and
electronic parameters, separately and in combination, in many different
frequency ranges, and to varying degrees of accuracy and precision. You
may know exactly what you want to measure and the conditions of measurement,
yet there is no quick way to narrow your selection to those instruments
that qualify.
Certain so-called "buyers' guides" try to classify instruments,
but the task is too much for them. Several manufacturers publish excellent
catalogues that bring order to their own houses. But the job of classifying
all instruments in terms of well-defined standards remains.
A hopeful beginning is the recently published program of IEEE Subcommittee
25.1 on Basic Standards and Calibration Methods. As a first step, this committee
has designated three echelons of accuracy level. Echelon I is the highest
calibration accuracy available within a country, Echelon II an intermediate
level, typically that of the calibration laboratory of an instrument manufacturer,
and Echelon III a level at which measuring instruments are calibrated before
use by the ultimate consumer. For each echelon, various IEEE Technical Committees
will report on the measurement accuracies available and needed. In its statement
of objectives, the IEEE Committee says, "An appraisal of the present
status of electrical measurements points up mainly the limited availability
of complete, reliable technical information." All who make, buy, or
use electronic instruments will wish this particular committee Godspeed
in its work.
Measurement of Frequency
Today's most popular frequency-measuring instrument the counter--scarcely
existed ten years ago. Now that digital counting techniques are being
widely applied to so many measurements, the frequency counter must be
considered the most important new instrument of the past decade. The modern
frequency counter represents a unique melding of borrowed technologies:
the flip -flop circuit from atomic research, pulse techniques from radar,
binary switching logic from computers. The counter became a practical instrument
with the development of a method of converting binary information to
decimal, new digital indicators, and improved switching devices.
The principles of counters are well known, and are here worth only passing
mention: Successive cycles of the frequency to be measured are passed through
a "gate" and counted, the total being indicated by a digital display.
The counting time, or the time that the gate is open, is determined by a
reference crystal oscillator, usually built into the counter.
If the gate is set to remain open for one second, the digit display is
direct-reading in cycles per second. The unit of time is established by
a crystal oscillator--usually called the "time base"--and the
accuracy of this time base determines, more than any other single factor,
the accuracy of frequency measurement.
If one is interested chiefly in accuracy, then the significant specification
is the accuracy and stability of the time-base oscillator. The possible
error corresponding to time -base inaccuracy must be added to the plus -or
-minus one-count error inherent in all counters. Other errors can be caused
by noise -, but these are harder to evaluate.
Frequency ranges of counters vary widely, but the upper limit for direct
-reading counters is presently about 50 mc.
Heterodyne converters extend this range up to 1000 mc. with no sacrifice
in accuracy, and transfer techniques are used from 1000 Inc. to above 10,000
mc.
One of the most valuable features a counter can offer is versatility -the
ability to rearrange its components for different types of measurements.
Thus many firms advertise "universal" counters. This usually means
that the instrument can measure not only frequency, but also period, time
interval, and frequency ratio as well.
To pleasure period, the functions of the "unknown" and time -base
signals are interchanged, so that successive cycles of the unknown frequency
open and close the gate, while "clock" pulses from the time base
are counted. As a result, the digits displayed indicate the number of time
units (e.g., 10 -µsec. units if the time base is a 100-kc. oscillator) passed
during one cycle of the unknown frequency. At frequencies much lower than
that of the time base, a period measurement will yield greater precision,
due to the higher count used.
A time-interval measurement also counts "clock" pulses from
the time base, but here the gate is opened by one signal and closed by
another. In frequency-ratio measurement, one signal is used to open and
close the gate, while the other is counted.
A consideration of increasing importance is the adaptability of the counter
to accessory instruments. If you require permanent records of measurements,
you will need either a data printer or the combination of digital–to-analog
converter and analog recorder. Whether a particular counter will operate
with a certain printer or D/A converter depends on the type of coding used,
input and output voltage levels, and other considerations. Failure to check
these in advance can easily lead to a pair of incompatible instruments.
Digital counters are by no means the only way to measure frequency, and
it is unlikely that they will ever take over completely. For some applications,
in fact, digits are a real nuisance. Suppose, for example, that you are
trying to adjust a variable-frequency oscillator to exactly 12,230 kc.,
while monitoring the output frequency on a counter. You trim one way and
then the other, as the digits dance tantalizingly around (but not on) the
desired frequency. How you would long, at this point, for a heterodyne instrument
with which your ears could guide you quickly to zero beat! It is a good
idea to remember that any good variable -frequency reference, such as a
signal generator, can be used with mixer and detector to measure frequency.
At frequencies up to about 1.5 mc., there are lab analog instruments available.
One, using an interpolation technique with most of the frequency suppressed
onto a switch setting, offers an over -all accuracy of ± 0.2% from 3 cps
to 1.5 mc.
And instruments for coarse frequency measurements–wave-meters and grid-dip
meters--still enjoy a brisk market.
There is every reason to expect further substantial improvements in frequency
measurements. Accuracy is, as mentioned, largely a function of the accuracy
or stability of the frequency reference. Thus, it is possible to increase
the accuracy of any counter by the use of a highly accurate frequency standard
as the time base.
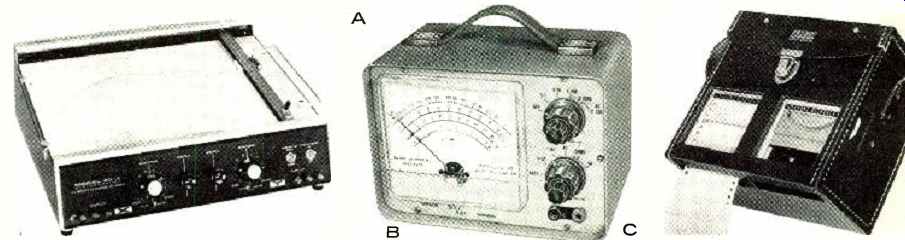
------ (A) Graphic recorders are widely used in industry where a written
chart showing one or more variable functions is required. The recorder
shown, a Moseley 2D-4, draws rectangular coordinate curves from two related
sources of d.c. electrical information on standard graph paper. Writing
area is 10 x 15 inches. High -gain solid -state servo amplifiers drive the
two motors that are coupled to balance pots and recording pen. Ten calibrated
input ranges from 0.5 mv. /div. to 10 v. /div. may be selected from the
front panel of the recorder. The liquid-ink pen has a maximum writing speed
of 15 inches per second. Price is $1490.
(B) Even
vacuum-tube voltmeters have become transistorized. DeVry transistorized
meter combines advantages of a v.t.v.m. with those of a sensitive microammeter
for service -bench use. Instrument is completely portable, operating
on four flashlight batteries. It has usual a.c., d.c., and resistance
ranges, along with four d.c. current ranges from 50 ua. to 50 ma. full-scale.
D.c. input impedance is 10 meg on most ranges. The price of the voltmeter
is $89.50 ready -wired or $64.50 in kit form.
(C) Graphic recorders need not be large and bulky as shown by the Amprobe
ATM -2. This is actually two most -frequently used recorders in a single
compact carrying case. One of the units is an expanded range a.c. voltmeter
and the other is a high -current a.c. ammeter. Roll of 21/2" wide pressure
-sensitive paper, traveling at 12" per hour carries the record of voltage
and current. With the paper removed, pointer position is visible on a calibrated
scale so that unit can be used as an indicating meter. Price of the 2recorder
unit and case is $181.20.
Measurement of Capacitance & Inductance
Capacitance and inductance measurements involve similar techniques and
limitations. The basic instrument for precision measurements is the bridge--an
instrument that permits the adjustment of a calibrated known until a state
of electrical balance exists between it and the unknown. Here, as in frequency
measurements, the major burden is on the accuracy of the "calibrated
known," or standard. But even the best standard is of little practical
use in measurements unless its accuracy can be somehow extended over a wide
range of values. One significant advance in bridges over the past few years
is the transformer ratio -arm bridge, a device for extending the usefulness
of a single standard over a range from 1 /1000 to 1000 times its value or
better, with no sacrifice in accuracy. Although the transformer bridge was
originally conceived over 30 years ago, its principles have only recently
been exploited in commercial instruments. The most accurate capacitance
bridges available use ratio arms to achieve direct reading accuracy of 0.01%
over a wide range of capacitance.
Capacitance standards can be made more accurate than inductance standards.
The capacitance bridges just mentioned use ±0.005% standard capacitors,
for example, whereas the best standard inductors are ±0.1% units. Inductance
bridges therefore use capacitors, rather than inductors, as standard reactances.
The most accurate inductance bridges available offer ± 0.1% direct -reading
accuracy.
The ultimate accuracy of most precision bridges is, incidentally, well
beyond the direct-reading accuracy. A high degree of resolution, that is,
a readout of five, six, or more significant figures, can be used for ultraprecise
inter-comparisons. One of the 0.1% capacitance bridges, for example, permits
inter-comparison of capacitors to within one part per million.
Capacitors and inductors can be measured in terms of either series or parallel
equivalent circuits, and many bridges therefore offer switch selection of
C,, Cn, Ls, and Lr. The difference between Ls and L and between C. and Cr
is less than 1% for inductors with "Vs" over 10 and for capacitors
whose dissipation factor is less than 0.1.
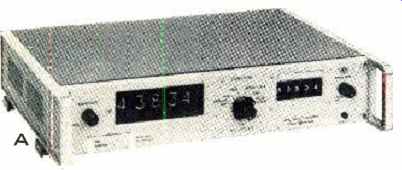
----- (A) Another example of an electronic counter is the Hewlett-Packard
52141.. This instrument totalizes and measures frequency and period.
In addition, it can directly display readings in practical units, such as
gallons /sec. or ft. /min. from appropriate transducers. Two sets of decades
are used: one to register the signal being counted; the other may be preset
to any number from 1 to 100,000 to control a gate circuit that may be used
to operate other equipment. Unit can be used to monitor automated processes,
and for production testing and for laboratory applications. The price of
the electronic counter is $1475.
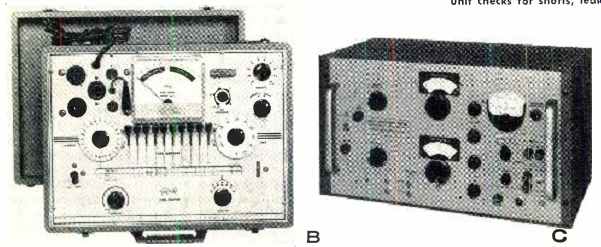
(B) There is still much equipment in use that employs vacuum tubes. To
check the performance of these tubes on the service bench requires an
easy-to-use, flexible tube tester, such as the Triplett 3414 shown. This
unit checks for shorts, leakage, and plate conductance. A self-contained
roll chart supplies test setup data. Sockets are provided for octal, loctal,
and miniature tube types. The price of the tester is $109.50.
(C) An example of direct
-reading, accurate capacitance bridge that can measure very small capacitance
values is the Boonton Electronics 75B shown. The instrument was originally
designed for use in measuring the temperature coefficient of small "zero
temperature coefficient" ceramic capacitors. It is also used for capacitance
measurements of semiconductors at millivolt levels. Lowest range of the
bridge is the extremely small value of only 0.1 pf. full-scale. Values as
high as 1000 pf. can be measured with this particular capacitance bridge.
Price: $1375.
Resistance Measurements
The instruments most often used to measure resistance are the ohmmeters
included with many voltmeters. The accuracy of such an ohmmeter is limited
to 5 or 10 %, partly because of trying to squeeze an infinite range into
a finite scale.
The resistance bridge is easily able to achieve 0.1% accuracy up to 10
megohms or so. Measurements of very high resistance are made by megohm
bridges, which supply the required high voltages. One megohm bridge will
measure resistances up to 10^15 ohms, with 1% accuracy up to 10^15. Resistance
limit bridges are direct-reading in percent deviation rather than in
ohms. Such bridges are used by production-line personnel to determine
quickly whether a component is within specified tolerance.
The exceptional accuracy of frequency standards has been applied to the
measurement and standardization of other quantities. The ohm has thus been
defined in terms of frequency and length, and standard resistors can now
be specified in terms of a few parts per million. Standard resistors are
used in series and parallel combinations, linear voltage dividers, and conventional
bridges to extend their usefulness over a wide-range of measurement. Present
state-of-the-art for general-purpose, wide -range resistance bridges seems
to be the same as for capacitance: ±0.01 %. The most popular bridges are
those that measure resistance, capacitance, and inductance--the so-called "universal" bridges.
This popularity is easy to understand when, in this day of four and five
-figure prices, less than $500 can buy a self-contained, battery-operated,
portable, five bridges–in-one instrument that measures C, R, and L to ±
1 %, and also measures dissipation factor and "Q."
Voltage
Measurement
The digital revolution came to voltmeters less than ten years ago, and
digital voltmeters are now produced by many manufacturers. The extra precision
afforded by digital readout is of especial advantage in d.c. measurements,
where the attainable accuracy warrants such fine resolution. Digital voltmeters
are considerably more expensive than the usual analog meters of comparable
ranges and quality, and this relation is likely to continue. Another relation
that seems basic is that between the accuracies attainable in a.c. and d.c.
measurements. While d.c. voltage can be measured to about ±0.002 %, the
best a.c. measurements are at least an order of magnitude less accurate.
The a.c. voltage is usually measured in terms of a d.c. equivalent, and
the translation from one to the other has to cost accuracy.
The scales on almost all a.c. voltmeters are calibrated in terms of r.m.s.
voltage. However, until a year or two ago, most voltmeters actually responded
to either peak or average value, with the scale conversion in terms of r.m.s.
voltage assuming sine -wave input. Now “true r.m.s." voltmeters have
begun to appear in quantity. The most common type of true r.m.s. voltmeter
measures a.c. voltage in terms of its heating effect on a thermocouple.
This effect is compared with the heating effect produced by a reference
voltage. Other true r.m.s. meters use dynamometers and rectifiers whose
non linearities synthesize r.m.s. response. True r.m.s. response cannot
be maintained by these means for all input waveforms, and the specification "maximum
crest factor," that is, the maximum ratio of peak to r.m.s. voltage
for which the voltmeter is reliable, is especially significant in pulse
work.
The peak- and average-responding voltmeters are highly refined from years
of development, and will probably continue to enjoy considerable popularity
based on their price advantage.
Most peak instruments rectify the input voltage and then amplify the d.c.
Their chief advantage is good high -frequency response. Also, since they
include a d.c. amplifier, they can be made to measure both a.c. and d.c.
voltage quite easily.
A disadvantage at low voltages is that the a.c. output no longer bears
a linear relation to the a.c. input.
The typical average-responding voltmeter first amplifies the input signal,
then rectifies it. A wide -band amplifier, stabilized by negative feedback,
is generally used. Although its high-frequency limit is well below that
of the peak-responding voltmeter, it can measure much smaller voltages.
At very high frequencies, broadband amplifiers are difficult to design.
At low voltage levels, rectifiers assume complicated characteristics.
Therefore, the measurement of high frequency, low-level voltages poses a
special problem. One method of solving it is to accept the complex rectifier
behavior and compensate for it on the meter scale. Another is to use a matched
pair of semiconductor rectifiers, one to rectify the input voltage, the
other to rectify a low-frequency reference voltage, and to compare the d.c.
outputs. Commercial instruments of both types are available.
The measurement of very small d.c. voltages is best accomplished by an
electrometer. Some electrometers convert the small d.c. voltage to an
a.c. voltage that is amplified and metered, while others use direct -coupled
amplifiers. A good electrometer can measure d.c. voltages down to a few
microvolts or less and currents to below 100 femto-amperes ( a femto-ampere
is equivalent to 10^-15 ampere) .
Wave Analyzers & Graphic Recorders
Many voltmeters travel under other names, because they are only indirectly
concerned with mere measurement of voltage. An example is the frequency-selective
voltmeter known as a wave analyzer. Operating ahead of the voltmeter in
a wave analyzer is a filter that passes only a certain frequency band. The
bandwidth is a constant number of cycles in some analyzers, a constant percentage
of center frequency in others. The constant -cps bandwidth is generally
more useful except at very low frequencies or where a logarithmic plot is
required. Optimum bandwidth depends on how much detail you need. Very narrow
bandwidths give more data but require more time for analysis. Wider bandwidths
offer greater convenience at the cost of some detail. A wave analyzer with
switch selection of two or three bandwidths lets you have your cake and
eat it, too.
Of course, the chief application for wave analyzers is waveform measurement,
and thus the oscilloscope and graphic recorder are important accessories.
The combination of a wave analyzer and an oscilloscope or recorder is known
as a spectrum analyzer. Spectrum analyzers are available from very low frequencies
to at least 100,000 mc. Most use automatic frequency sweeping to give continuous
oscilloscope display. The modern spectrum analyzer combines the features
of the wave analyzer, oscilloscope, and sweep generator in a sophisticated
system extremely useful not only in electrical waveform measurements, but,
with transducers, in studies of sound, vibration, heartbeats, and analogous
phenomena.
The graphic level recorder is another type of voltmeter. A pen marks the
voltage level on a piece of graph paper.
Then, as the paper is moved under the pen, with the motion of the paper
corresponding to changing time or frequency, the pen continues to plot the
voltage level. The result is a response plot of the input voltage vs frequency
or time.
The graphic recorder is widely used for spectrum analysis. Although it
can't keep up with the sweeping rates of an oscilloscope, it has its own
advantages, principally the permanence and greater resolution of its record.
Graphic level recorders measure a.c. or d.c. voltages. There is a wide
variety of chart speeds, writing speeds, and voltage and frequency ranges.
Some write with ink, some electrically. When the recorder is used with
a variable-frequency source, a primary consideration is the means for transferring
the frequency scale onto the chart paper. Some means of linking the oscillator
frequency dial to the recorder drive mechanism must be provided, as well
as chart paper especially calibrated for the setup.
Microwave Measurements
At microwave frequencies, where circuit dimensions approach wavelength,
measurement techniques often differ from those at lower frequencies.
The importance of physical dimensions makes partners of the development
engineer and the machinist, especially at the higher microwave frequencies
... where waveguide is employed to replace coaxial line.
Power Measurement. Voltage and current vary widely along a transmission
line at microwave frequencies, and power becomes a fundamental quantitative
measurement. In microwave power meters, the power is converted into heat
and measured either in terms of temperature rise or of resistance change
in a bolometer. The first type the calorimetric power meter--is generally
used for measurements above 10 watts, while bolo-meters are limited to lower
levels.
Frequency Measurement. The effects of physical dimensions at microwave
frequencies are used to advantage in several instruments unique to the microwave
part of the spectrum. The cavity type frequency meter is the microwave version
of lower-frequency tuned-circuit wave-meters. The cavity is a simple wave
guide or coaxial section that can be sharply tuned to resonance. The signal
to be measured is coupled to the cavity, the cavity adjusted, and the resonant
point noted on a finely calibrated scale.
The mechanical nature of such a device limits accuracy to about x-0.01
%; for higher-accuracy measurements, multipliers, mixers, and transfer
devices are added to lower-frequency precision instruments.
V.S.W.R. and Impedance Measurement. Another important instrument peculiar
to the microwave region is the slotted line. The slotted line is a section
of coaxial line or waveguide that is slotted, with a movable probe sampling
the field in the line. The slotted line can hardly be considered a direct-reading
instrument; like the cavity frequency meter, it is simply a device for "coupling
into" a transmission line to sample the standing -wave pattern. But
whereas the cavity includes a calibrated frequency scale, the results of
the slotted -line measurement ( v.s.w.r., impedance, and associated parameters)
are usually taken off a Smith Chart, slide rule, or auxiliary detector.
The admittance meter and reflectometer are alternate instruments for the
measurement of impedance, v.s.w.r., etc. The admittance meter uses a bridge
technique, in which pickup loops in the bridge arms are oriented to balance
the unknown admittance against conductance and susceptance standards. The
reflectometer is basically a power meter used to measure the ratio of incident
to reflected power, or reflection coefficient.
There have been few changes in the fundamentals of microwave measurement
over the past decade. Of course, the frequency range of interest is always
expanding, and the instrument developer must keep pace. But the old bolo
meter and calorimeter still reign supreme in power measurements, even
though they are now associated with the greater convenience of self-balancing
bridges.
There is constant improvement in measurement accuracy throughout the spectrum,
but one cannot expect the same accuracies at microwave frequencies as at
100 kc. Once you have entered the gigacycle area (1 gigacycle = 1000 mc.),
you no longer talk about parts per million, and are very happy to measure
within parts per thousand. A tenth of a percent is a very decent accuracy
for a cavity frequency meter at 15 gc., and commercial microwave power meters
are typically in the 3 to 5% class.
REFERENCES
1. "A Program to Provide Information on the Accuracy of Electrical
Measurements," Proceedings of the IEEE, Vol. 51, No. 4, April 1963.
2. McAleer, H.T.: "Digits Can Lie," General Radio Experimenter,
Vol. 36, No 12. December 1962.
|