(source: Electronics World, Aug. 1963)
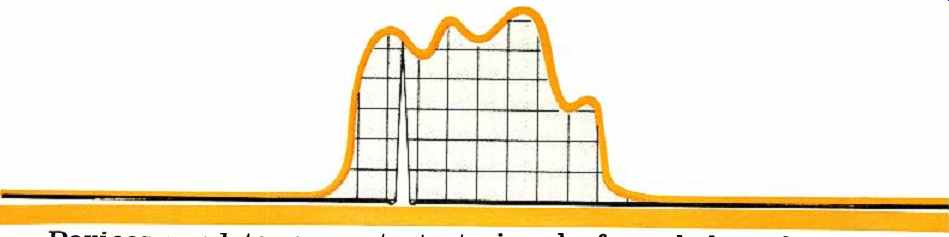
By HOWARD L. ROBERTS / Hewlett-Packard Co.
Devices used to generate test signals from below the audio range
up through microwaves. Performance, specifications, and effect of semiconductors
on equipment design are covered.
SIGNAL-generating equipment comprises a wide variety of electronic instruments
for generating test signals.
This class of instruments includes everything from the human finger, placed
on a grid connection, to complicated check-out equipment which automatically
generates a number of frequencies, voltages, and other signals for quickly
testing intricate missile systems.
The finger test may be all that you need if you merely want an indication
of whether the equipment is working or not. But when you need to know how
well the equipment works, then the appropriate piece of signal-generating
equipment must be called upon to provide the answer.
If, for instance, you want to measure distortion in an audio amplifier,
you need an oscillator that generates sine waves with far less distortion
than you expect the amplifier to have.
If you want to measure the rise time of a switching circuit, you ought
to have a pulse generator that produces pulses with rise times faster than
the switching circuit.
Types of Equipment
Signal-generating devices may be loosely grouped into two categories: special-purpose
and general-purpose generators.
Special-purpose generators are designed for specific kinds of tests and
usually generate only those combinations of frequencies needed to test
certain kinds of equipment. An example of this type is an FM stereo test
generator. Special-purpose generators are best suited for making repetitive
measurements since their built-in features make specific tests faster.
General-purpose equipment may not have many combinations of signals but
usually can generate signals over broader ranges of frequency and amplitude.
These generators find most use in the experimental and development laboratory,
both professional and hobbyist, but are often used for specific tests as
well. Presumably, any test performed by a special purpose generator can
be performed by an assortment of general-purpose equipment, but several
instruments may be required to do the same job.
Since the basic design principles involved in general-purpose laboratory
instruments are also used in special-purpose generators, we'll confine this
discussion to general-purpose instruments.
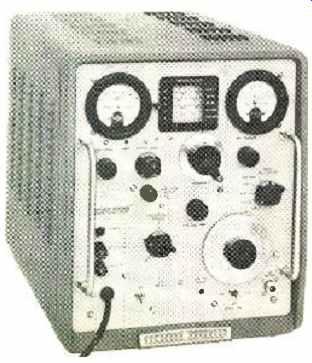
--- When an accurate, stable v.h.f. signal source is required for receiver
or v.h.f. amplifier measurements, then a standard signal generator is
required. Our example is the Hewlett -Packard 608D. The instrument is especially
suited for precise tests of narrow -band v.h.f. aircraft communications
equipment.
It has a calibrated output of 0.1 ¡iv. to 0.5 v. throughout the 10 to 420
-mc. band. Output frequency can be set to within a few kc. with built -in
crystal calibrator. Price: $1300.
Signal-generating equipment is often grouped according to the shape of
the output signal waveform. Sine-wave generators form one important category
since the sine wave is the most fundamental waveform. These instruments
are widely used to test equipment performance at discrete frequencies.
Oscillators and Signal Generators
Among sine-wave generating equipment,
a distinction is made between oscillators and signal generators. A
signal generator is an oscillator that has accurately known amounts of output
power as well as known frequencies. In many cases, this means simply that
a signal generator has a meter to show the power generated by the output
stage, and a precision attenuator for cutting down the indicated power by
known amounts.
At audio frequencies it is a simple enough matter to read oscillator output
with a lab voltmeter. For this reason, output meters are not included in
the majority of audio oscillators. At r.f. frequencies, though, voltage
measurements are more complex so that r.f. generators include a built -in
output meter designed especially for each generator. (An r.f. generator
without a meter is known as a "signal source.") RC oscillators
have dominated the audio and neighboring ranges for more than twenty years.
This range extends from subsonic through ultrasonic and into the low-frequency
r.f. ranges.
RC oscillators are so named because their frequency-determining networks
consist only of resistance and capacitance--no inductance whatever. The
reason for using an RC circuit is that if an LC circuit were used, the large
inductors required for low-frequency oscillations would be much too cumbersome.
The RC oscillator is much lighter, easier to build, and, as it turns out,
a good source of distortion -free sine waves.
These oscillators generally use some form of amplitude limiting other than
driving a tube to cut-off and /or saturation.
The most common form of amplitude control is the use of a small incandescent
lamp as a non -linear resistance in one of the feedback loops. The lamp's
resistance changes, if the oscillation amplitude changes, readjusting
the amount of feedback to counteract the change in amplitude. This type
of limiting prevents the tubes (or transistors) from being driven beyond
their linear operating regions and accounts for the low distortion output
of these oscillators.
The oscillator circuit may also be followed by a tuned amplifier -filter
ganged to the tuning dial so as to track the oscillator tuning. Filtering
attenuates what little harmonic content there may be in the oscillator
signal, resulting in hyper-pure sinewave output. This type of instrument
is most useful for making distortion measurements on other types of equipment.
Multivibrators with very long time constants are used in instruments for
the very low frequencies, some of these operating as low as 0.001 cps (
about 1 cycle per 15 minutes!) . Multivibrators generate square waves, of
course, but the square waves are changed to sine waves by waveshaping networks.
This is just opposite to the audio -range sine /square wave generators which
start with sine waves and form these into square waves with clipping or
triggering circuits.
Above 1 mc., the usual RC oscillator runs into certain problems. The tuning
capacitors are in a range of values comparable to distributed circuit
capacitance which makes tuning less precise. Phase-shift oscillators, a
different form of the RC oscillator, swamp out the stray capacitances and
can be used up to 10 mc. and beyond.
Beat -frequency oscillators are also used throughout the low frequency
region. These instruments use two r.f. oscillators, one generating a fixed
frequency and the other being tunable.
The outputs of these oscillators are mixed and the difference frequency
is separated from the mixture by filter circuits.
The advantage of the beat -frequency oscillator is that very wide frequency
ranges can be covered by a single sweep of the tuning dial, at the expense
of tuning resolution however. The disadvantage is that the oscillators must
be made many times more stable than the desired stability of the output
signal because of the large step -down in frequency. High quality beat -frequency
oscillators made for laboratory applications do achieve the desired stability,
however.
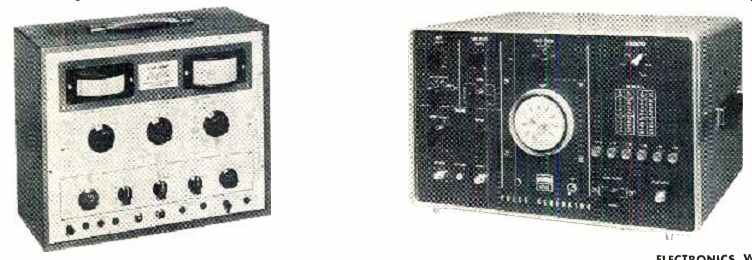
------ Designed mainly for servicing AM, FM, and TV receivers is the
Hickok 288AX sweep and marker generator. The instrument has 8 bands of
unmodulated or AM signal output from 35 kc. to 110 mc. as well as frequency-modulated
output up to 160 mc. A built-in audio oscillator, tunable from 20 to
15,000 cps, is used for modulation or for audio tests. A crystal calibrator,
with a choice of 100or 1000 -kc. signals, is also provided along with a
3-range decibel meter. Price of this unit: $315. [right] An example of
a versatile pulse generator is the Fairchild DuMont 404-B. This device can
generate pulses suitable for use in signal-sampling techniques in conjunction
with oscilloscopes. Another application is to trigger scope sweeps at the
proper time to view the leading edges of very short transients. Pulses can
be generated with widths continuously variable from 0.05 to 105 psec., with
various delays, and with repetition rates up to 250,000 p.p.s. Generator price:
$760.
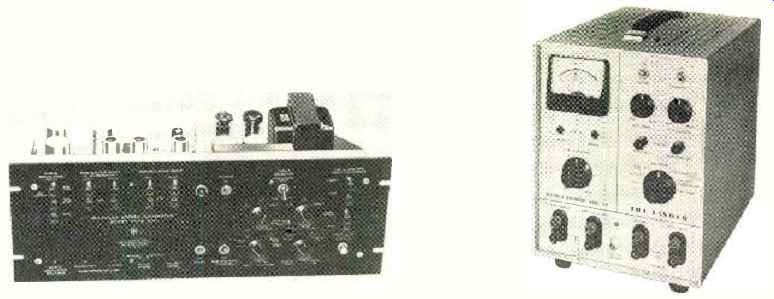
----- [LEFT] A multiplex stereo generator developed for design and production
testing of FM multiplex adapters, tuners, and receivers is the Scott
830. The unit has also been used by FM broadcast stations to produce a
composite stereo signal. When feeding a composite stereo signal into the
front end of a tuner, the generator's output is used to modulate a laboratory-quality
standard FM signal generator. The price of the unit: $600. [RIGHT] Intended
mainly for the service technician working on FM multiplex equipment is
the Fisher Model 300 multiplex signal generator. The instrument is also
suitable for use in production testing. Incorporated are an audio oscillator
and an r.f. generator, which is factory tuned to the approximate center
of the standard FM broadcast band. An output level indicator is also provided.
Price of generator: $495.
R.F. Signal Generators
The designation "r.f." is another all-embracing term, originally
referring to signals that were destined to be radiated or detected as radio
waves. As it turns out, radio signals are now propagated at frequencies
even lower than 20 kc. ( standard frequency broadcasts such as WWVL) and
at super high frequencies measured in thousands of megacycles ( giga-cycles
) . Our discussion here will be confined mainly to those radio-frequency
generators which have lumped LC circuits, using coils and capacitors in
the resonant circuit.
During the forty-odd years that these generators have been used, they
have undergone considerable refinement. Factors which upset frequency
stability have been designed out of the circuits. Manufacturers have learned
how to wind coils tightly of low expansion wire on matched ceramic forms
so that there is little change of inductance with a change in temperature.
In high-quality generators, capacitors are made from rigid precision -machined
plates, which are silver- or gold-plated for minimum resistance to r.f.
surface currents (skin effect). In recent years negative feedback has been
used in various ways to improve amplitude stability and modulation characteristics.
Even with all these refinements, a good r.f. signal generator still includes
an accurate crystal-controlled frequency calibrator to enable precise
setting of frequency. The calibrator frequency is usually a whole number,
such as 1 mc., so that it is easy to spot the harmonics.
Such r.f. generators also include amplitude or frequency modulation capabilities.
This enables the generator to supply signals which resemble the actual signals
to be used by the equipment being tested.
When the response of a sharp cut-off filter or i.f. circuit is being measured,
modulation may wobble the r.f. frequency across the circuit's cut-off point,
broadening the apparent response curve. For this reason, most r.f. generators
use the master oscillator-power amplifier (MOPA) configuration which isolates
the oscillator from the output amplifier, permitting amplitude modulation
of the amplifier without affecting the frequency of the oscillator.
The output impedance of r.f. generators deserves special comment. If the
output cable and load impedances aren't the same, r.f. energy reflects
from the load, giving rise to standing waves. If the generator itself is
not matched to the output cable, then reflections are re-reflected, which
alters the character of the standing wave and interferes with measurement
accuracy. Most r.f. generators are matched to one of the 50, 75, or 300-ohm
impedance levels commonly used but pads or baluns are required to match
other impedances.
Because of standing waves, the r.f. voltage at the generator may be different
from the load voltage. For this reason, an output voltmeter is a reliable
indicator of signal strength only when the load is matched to the generator.
The attenuators of r.f. generators require careful design to account for
distributed capacitance and inductance. These devices are frequency -sensitive
unless components are positioned carefully. Furthermore, the impedance of
the attenuator must be matched to the output cable at all settings.
U.H.F. and Higher Frequencies
Above .300 mc. the inductance and capacitance of tuned circuits become
so small that it is difficult to treat them as discrete circuit elements.
At these higher frequencies, resonant cavities Of one sort or another are
generally used as the resonating elements.
Gridded tubes for u.h.f. frequencies have rather odd shapes so that all
elements make contact at the right place when installed in a cavity. Above
1000 mc., klystrons are most often used as oscillators.
Microwave signal generators use a single power oscillator for the r.f.
channel, avoiding the cost and complexity of a power output stage. Because
of this, modulation is usually restricted to pulses and square waves. Modulating
waveforms turn the power oscillator completely off and on, making the transition
between off and on states as fast as possible to minimize frequency pulling
or FM as the klystron voltage changes. On the other hand, it is relatively
easy to frequency modulate a klystron over a range of a few megacycles by
applying a saw-tooth voltage to the klystron repeller.
The attenuators of these generators also avoid the use of lumped circuit
elements, the waveguide-beyond-cut-off type being favored. This attenuator
uses a pickup loop which slides in and out of a length of waveguide that
opens into the resonant cavity. The waveguide dimensions are smaller than
those required to carry an electromagnetic wave at the frequency of the
oscillator but the cavity's magnetic field leaks into the waveguide. The
further the loop is from the cavity end, the weaker the field is and, what
is more, the field strength drops off in a predictable manner so that these
attenuators are inherently accurate.
Sweep Generators
Sweep generators are indispensable for anyone concerned with circuit response
throughout a band of frequencies. A straightforward way of obtaining a sweep
frequency is to add a motor drive to the oscillator tuning dial. This approach
is widely used at audio and low frequencies, where electronic techniques
for changing frequency fail to achieve the necessary wide (in terms of number
of octaves) bandwidth. The beat -frequency oscillator shines here as a means
of getting wide sweeps.
Motor drives are also used at radio frequencies but vibrating capacitors
often serve to vary the oscillator frequency.
At microwave frequencies, the voltage -tuned backward -wave oscillator
(b.w.o.) can easily sweep through an octave band of frequencies simply by
modulating the helix voltage.
Motor-driven sweeps, unless carefully designed, cause mechanical vibrations
which induce microphonics that generate spurious oscillations. Motor -driven
sweeps are also limited in speed. Electronic sweeps, which vary the oscillator
tank circuit's resonant frequency either with a reactance tube, a voltage-variable
capacitor, or a saturable reactor, are used wherever possible.
Many service-shop sweep generators have a fixed sweep rate of 60 cps,
taken right from the power line. This simplifies circuitry and also makes
synchronization of related oscilloscopes easier.
Circuit response is usually displayed on an oscilloscope.
The sweep rate should be fast enough to obtain a flickerless display. Slow
sweep rates are required for pen recorders which may trace with more detail
than a scope does.
Pulse and Square-Wave Generators
Pulse and square-wave generators produce signals of an "off-on" nature
by switching between two d.c. levels. These generators are used to check
systems which operate in a pulsed mode, such as radar modulators, oscilloscope
triggering circuits, computer circuits, and teletypewriter systems.
These generators are also used for transient response testing of linear
systems.
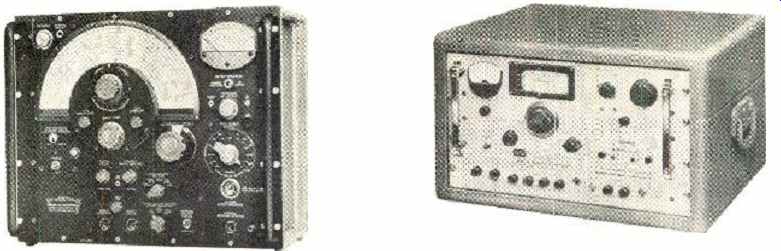
---------- In order to take quantitative r.f. frequency response measurements,
a sweep frequency generator is used. The response curve, traced on a
scope, is usually marked with a marker generator. Incorporating both
functions in a laboratory instrument is the General Radio 1025 -A. The
unit covers 0.7 to 230 mc. plus two bandspread ranges (400 - 500 kc.,
10.4 - 11 mc.) for i.f. curves. The accurate marker pip is continuously
adjustable in frequency and amplitude. Price: $3250. [RIGHT] Another
example of sweep frequency generator is the Jerrold Model 900 -B. This instrument,
which has been designed for laboratory or production use, generates sweep
signals with center frequencies from 500 kc. up to 1200 mc. in the u.h.f.
range. Sweep widths vary from a narrow 10 kc. to a wide 400 mc. for the
higher frequencies. The unit also has built-in crystal-controlled harmonic
markers and provision for external variable marker signal generator.
Price of unit: $1980.
The shape of the input pulse or square wave has to be neatly rectangular
if anything is to be learned from the shape of the output pulse. Rounding
of the pulse corners, or overshoot, wiggles, and sag of the pulse top, are
the symptoms of system performance so should not be present on the input
pulse that is produced by the pulse generator employed.
A square -wave generator is really a pulse generator having equal "on" and "off" times. "On" time
duration therefore varies with frequency. Pulse generators have one control
for frequency, or pulse repetition rate, and a separate control for "on" time
duration, or pulse width. "On" time is usually much shorter than "off" time
so that pulse generators are often used to drive r.f. oscillators or other
circuits where high power is needed during the pulse but where average power
should be kept low.
These generators usually use some form of multivibrator circuitry for wave
generation with clipping circuits and other devices for "squaring up" the
waveform. An exception is the mercury -wetted relay which, unlike ordinary
relays that have a certain amount of contact bounce, latch on for good when
contact is made. Generators using these relays are able to generate high
power pulses with turn -on times (rise times) measured in fractions of a
nanosecond (10-° second) but their use is limited because of relatively
slow repetition rates.
Noise Generators
Measuring signal-to-noise ratios in receivers with sine waves or other
coherent signals is complicated because of theoretical considerations involving
bandwidth and noise power per cycle bandwidth. This difficulty is circumvented
by using broadband noise from a suitable generator as the test signal. A
comparison of receiver noise output without signal input to noise output
with a known amount of noise power at the input, provides a measure of receiver
performance.
Noise generators are also used as a source of broadband signals. Since "white" noise
is an equal mixture of all frequencies, a test using a noise voltage as
the input is a test which covers the complete range of a system all at once.
This is a good one to use for spotting any resonances in audio systems or
in acoustic measurements.
Broadband noise sources require careful design; otherwise, resonances may
prevent an equal -energy distribution for all frequencies. Noise sources
include hot resistors, gas tubes, and temperature limited diodes (these
are diodes operated with low filament voltages and with plate voltages high
enough to maximize shot noise in the diode current). Impact of Semiconductors
Transistors are used in signal generating equipment where their low power
drain and low heat can contribute to a lightweight, battery -powered design.
As the cost of transistor circuitry becomes more competitive with vacuum
tubes, we can expect to see more use made of transistors in test equipment.
Low-capacitance semiconductor diodes can be used at much higher frequencies
than thermionic diodes and are used as harmonic generators to double
the frequency of microwave generators. They do this by partial rectification
which distorts the r.f. wave, creating harmonic frequencies.
Tunnel diodes find application as low-level, low impedance r.f. generators
and as fast -rise pulse generators. One available pulse generator uses a
simple tunnel diode circuit to generate pulses with rise times of less than
0.1 nanosecond. Pulse amplitude, though, is less than half a volt.
The step-recovery or snap-off diode represents a recent discovery which
is being designed into fast-pulse generators.
Any semiconductor diode stores a charge, when forward biased, which flows
as reverse current for a brief interval when a reverse bias is suddenly
applied. The step-recovery diode is designed so that this stored charge
is used up abruptly, making the transition from short-circuit ( charge
carriers flowing) to high impedance (no charge carriers) in less than
a nanosecond. This device is used in a new pulse generator as a pulse
sharpener to square up the output pulses.
Still another kind of diode is revolutionizing the modulator in microwave
generators. This device, known as a p-i-n diode because of the intrinsic
(no impurities or doping)
silicon layer sandwiched between the p and n layers, also exploits charge
carrier flow. At microwave frequencies, a half-cycle does not last long
enough for the charge carriers to be swept out, so that current flows
through the diode in alternate directions in response to the r.f. voltage
waveforms.
The diode appears resistive, however, and the apparent resistance is changed
by varying the diode bias.
A voltage -controlled attenuator is made by placing p-i-n diodes across
a transmission line so that they bypass or absorb some of the r.f. energy,
the amount that is bypassed being controlled by the diode bias. The oscillator
can then operate continuously and modulation is achieved by absorbing
various amounts of r.f. energy without causing reflections on the transmission
line.
With these diodes, it is now possible to amplitude modulate a klystron
output with sine waves, or any kind of complex wave for that matter. What
is more, the microwave power can be turned on and off more quickly with
these attenuators than power can be built up or damped out in high -"Q" cavities.
Equipment Accuracy
A quality r.f. signal generator built during the past ten or fifteen years
can be expected to have about a 1% or 2% accuracy in frequency setting.
Crystal-controlled calibrators provide a 100 times improvement at frequencies
corresponding to harmonics of the calibrator. This accuracy does not hold
at other frequencies, though, simply because the tuning dials of the instruments
cannot be read with that kind of precision.
However, frequency can be monitored with an electronic counter to a far
higher degree of accuracy. With the aid of a precision transfer oscillator
and heterodyning techniques, counters can determine frequencies as high
as 18,000 mc. /sec. (and higher) to an accuracy of 8 decimal places.
If an oscillator can be voltage controlled, phase -locking techniques can
be used to maintain the output frequency at the same stability as a reference
crystal oscillator. This can be done, though, only at spot frequencies corresponding
to harmonics of the crystal oscillator frequency.
Precisely known signal frequencies are available from frequency synthesizers,
which obtain various r.f. frequencies by dividing, multiplying, adding,
and subtracting other frequencies derived from crystal -controlled oscillators.
The newest signal source of this type generates frequencies all the way
from 0.01 cps to 50 mc. in , 0.01-cps steps by push-button control. All
of these 5 billion discrete frequencies are derived from one highly precise
1-mc. crystal oscillator and all are accurate to better than 0.0000003%!
This is three ten-millionths of one percent.
The accuracy of signal generator output amplitude indications can't approach
accuracies such as those available for frequency -primarily because highly
precise standards of r.f. power never existed until only recently. High-quality
generators have had output accuracies in the neighborhood of 1 to 2 db.
Now that more accurate r.f, power monitors are becoming available, the
trend is towards the use of power leveling for signal generator output
amplitude control. In these systems, the power monitor maintains constant
output power by means of a wide -band, direct -coupled feedback loop which
controls an amplifier control grid, or one of the new electrically controlled
p-i-n diode modulator/ attenuator units. Output power flatness can be held
to within 0.2 db with this technique. The accuracy of the power monitoring
system is typically ± 0.5 db overall, however an additional ± 1 db should
be added because of attenuator accuracy throughout the frequency range of
the equipment.
Little effort has been expended on controlling the accuracy of pulse generators
with respect to pulse width and amplitude; the major design effort being
directed towards achieving good pulse shape. Pulse generator calibrations
are used for no more than a rough initial indication of pulse amplitude,
width, or repetition rate. Because pulse generators are most often used
with an oscilloscope, the pulse generator controls are usually adjusted
exactly while monitoring on the oscilloscope.
Choosing Equipment
The first thing to do is to take a good, hard look at what the equipment
is intended to do. What frequency ranges are needed and which can be
omitted? What kind of signals are desired -sine wave, pulsed, FM, AM,
or what? What stability does the generator have? That is to say, if you
set it at one frequency how confident can you feel that the generator
will stay exactly at that frequency? What is the amplitude stability? If
you set the output for 1 volt r.m.s., will it stay at the exact 1-volt setting
all afternoon, or if you change frequency? A design engineer is very
much concerned with these matters. When designing an i.f. strip, for instance,
he needs a generator with an output that is flat across the band, so
that the detected response will show him where the i.f. strip isn't flat.
The serviceman repairing the same strip can get by with a generator of lesser
quality. About all that he can do is to set the traps to the right frequencies,
get about the right slope to the band edges, and take excessive tilt off
the passband. A few humps in the generator output won't affect these adjustments
significantly.
Check the performance of the attenuators, which are needed to prevent overdriving
high -gain equipment. Is the attenuator accurate, and is its attenuation
constant with frequency? And watch out for r.f. leakage. A big attenuation
factor doesn't tell you anything if r.f. power leaks into the tested circuit
anyway.
How much harmonic content is present if you are supposed to be getting
pure sine -wave output? If there are harmonics, you may think that a
filter circuit is passing a certain frequency when it is really passing
a harmonic. With r.f. generators, the standing wave of a harmonic may
be much higher than the fundamental, providing a false indication.
Certainly, the reputation of a manufacturer is of considerable importance.
Reputable manufacturers check the performance of many production units
and then set specifications beyond the widest deviations. Sometimes their
specifications may not appear spectacular, but with this kind of equipment
performance will always be better than claimed.
|