(source: Electronics World, Dec. 1971)
By DAVID L. HEISERMAN
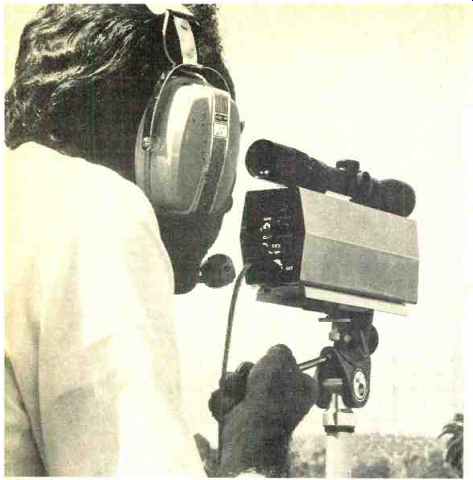
---This optical communicator, which transmits and receives voice or digital
information, is designed for "secure" point-to-point work.
With the r.f. spectrum so overcrowded, engineers are looking
to light portion of the band to handle short-range optical communications
links.
THE demand for additional broadband communications links is growing at
an ever-increasing rate, and there seems to be no end in sight. The problem
of overcrowded r.f. communications bands reached a critical point years
ago; but, until very recently, the FCC and the communications industry
have been at a loss for a truly satisfactory solution.
Development of the laser in the early 1960's gave communications technology
the breakthrough it needed to perfect the old idea of light-beam communications.
Unlike ordinary light, laser light can be multiplexed, heterodyned, beamed,
demodulated, discriminated, and otherwise treated in ways quite analogous
to microwave r.f. energy. But, unlike r.f. communications systems, optical
communications links (OCL's) cannot interfere with one another under ordinary
circumstances, and they are totally immune to both man-made and natural
r.f. interference. What's more, OCL's cannot interfere with existing r.f.
communications links.
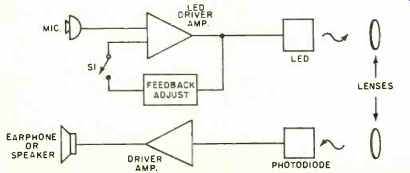
Fig. 1. Block diagram of an experimental LED optical transceiver. Devices
such as this promise to become quite popular among electronics and science
experimenters in future.
The extraordinarily high frequency of a laser-beam "carrier" lends
itself quite naturally to high-capacity broadband communications. A single
laser beam carrier, for instance, can handle several conventional TV channels,
three or four streams of high-speed digital data, some Picture-phone signals,
and hundreds of voice-grade channels--all with room to spare. Even the
relatively incoherent infrared light from inexpensive light-emitting diodes
(LED's) can carry several channels of TV information and computer data
on a single beam.
Optical communications, then, seems to be the ideal solution to the problem
of r.f. communications overcrowding.
However, optical communications is still in its infancy and few companies
can boast of an inventory of finished OC instruments. Dozens of companies
have developmental or prototype models in operation and information from
the R &D labs indicates that OCL's will be taking over a significant
portion of the r.f. communications overload by the mid-1970's.
At the present time, developmental work in optical communications falls
into two distinct categories: (1) versatile short-range systems using
laser diodes or LED's as light sources, and (2) long-range, high-performance
systems using conventional gas or solid-state laser sources. Devices in
the first category will eventually take over jobs now handled by radio
transceivers and short-haul cables and r.f. links. The bigger communicators
will handle long-haul, high-capacity tasks now performed by a wide variety
of commercial, private, military, and government communications systems.
Single-Channel LED Communicators
An LED emits light energy whenever current flows in a forward direction
through its p-n junction and the amount of light output is roughly proportional
to the amount of forward-biasing current. Most LED's, those made from
silicon-doped gallium-arsenide, emit a peak of 100 milliwatts of light
energy in the infrared portion of the light spectrum. Although LED light
lacks the coherence of laser light, it is quite pure compared to ordinary
incandescent light-a typical infrared-emitting LED has a spectral band
width of only 200 angstroms between half-power points. A fast response
time, on the order of 10 nanoseconds, also helps make LED's ideal sources
of light for short-range, intensity-modulated voice and data communicators.
LED modulator circuits are so simple and reliable that electronics buffs
are beginning to have a field day designing and building short-range LED
voice communicators.
Used with a set of 1-inch lenses or small telescopes, a LED transceiver
can operate over a range of about 250 feet in broad daylight and ten times
that far on a clear night. Not counting the optics, the parts for an experimenter's
intensity modulated LED transceiver runs between $15 and $25.
Fig. 1 is a block diagram of an experimenter's LED transceiver. Low-level
audio signals from the microphone go through a current amplifier circuit
that serves as a LED driver. Since the amount of light energy from the
LED is very nearly proportional to the amount of forward-biasing current
flowing through it, audio signals from the driver intensity-modulate the
output light energy. A lens following the LED focuses the light into a
narrow beam, thus increasing the effective transmission range.
Closing S1 completes a positive feedback loop around the LED driver amplifier.
The feedback-adjust circuit contains RC components that let the amplifier
oscillate at some fixed audio frequency-generally around 1 kHz. These
oscillations modulate the LED output and provide a convenient audio signal
for aligning the transmitter with a remote receiver unit. Once the operators
make the initial alignment, opening S1 stops the alignment tone.
A clever circuit designer will bias the LED and driver amplifier just
a bit below cut-off. Although this trick introduces some audio distortion,
it buys the user two advantages: (1) the transmitter, in effect, will
be voice activated and (2) it makes it possible to increase the LED's
peak output power. When there is no signal from the microphone the LED
and driver amplifier consume virtually no power, making it practical to
leave the transmitter in a standby mode at all times. Class-B current
pulses from the LED driver also allow the user to operate the LED in it
pulse mode and thus increase the peak output power.
The lens in the receiver section gathers in light signals from a remote
transmitter and focuses the energy onto a photodiode. The incoming light
signals modulate the photodiode's current, and a current amplifier boosts
the signal level to drive an earphone or loudspeaker. Slight cut-off bias
on the photodiode and amplifier can also make the receiver into a signal
activated device.
By using the infrared portion of the light spectrum, LED communicators
are relatively insensitive to most kinds of ambient light. Sunlight, unfortunately,
contains a great deal of infrared energy that tends to saturate the photodiode.
An external sensitivity control lets the operator adjust the cut-off
bias on the photodiode and thus cancel out the effects of stray infrared
energy when operating the system in broad daylight.
Isolating the receiver circuit from the transmitter circuits eliminates
the need for a send / receive switch. Optical isolation is much easier
to achieve than r.f. circuit isolation-the designer merely takes reasonable
care to make sure no light from the LED can reach the nearby photodiode.
One of the essential features of the simple LED transceiver just described
is that it modulates the light source directly and does not rely upon
some separate modulation device such as a vibrating mirror or voltage
sensitive optical filter. Directly modulated light sources, in fact, represent
one of the greatest advantages semiconductor light sources have over conventional
gas or solid-state laser sources.
In principle at least, just about every feature of the experimenter's
transceiver carries over to high-performance LED optical communicators.
The high-performance communicators might use better optical systems, higher
quality LED's and photodiodes, some schemes to get more power out of the
source, and extra circuitry such as a.g.c. and noise controls; but the
basic principles are the same for a hobby LED transceiver as for a six-mile
LED data link. Even the process of multiplexing a number of wideband channels
onto an LED beam is often more a matter of adding outboard electronics
than making any significant changes in the basic modulator and detector
schemes.
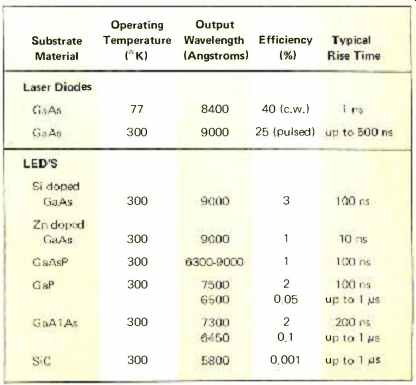
--- Laser diode and LED specs related to optical communications.
Multiplexed LED Communicators
The real advantages of optical communications come into play only when
users take advantage of the extraordinarily high information capacity
of coherent or near-coherent light. Although short-range, single-channel
voice or data LED communicators may become quite popular within the next
few years, the real R &D efforts are going into high quality, multi-channel
versions. Right now, three kinds of multiplexing schemes are getting the
attention of leading R &D labs: frequency, time-division, and spatial
multiplexing.
The most promising frequency-multiplexing scheme uses purely electronic
techniques to generate and modulate an electrical subcarrier for each
information channel. The sub carrier modulation may be AM, FM, or a combination
of the two. See Fig. 2. An electronic multiplexer circuit brings all the
channels together while a set of electronic filters minimizes any subcarrier
crosstalk. Only after going through all the multiplexing and filtering
processes do the signals go to a broadband LED driver and to the LED itself.
In theory, the only factors that limit the information-handling capacity
of this frequency-multiplexed LED communications system are the bandwidths
of the individual channels and the purity of the light source. In practice,
however, the limitations are due more to a lack of electronic devices
and know-how needed to take full advantage of a 30,000-GHz carrier.
These frequency-multiplexed LED communicators are among the most popular
OC devices in use today. A group of researchers at the University of Colorado,
for example, has developed a frequency-multiplexed LED communicator now
used by a CATV firm to carry TV signals from a mountain-top microwave
antenna to a city in the valley below. The system has a range of six miles
and it completely eliminates the need for stringing cables down the rugged
mountainside. This particular system, by the way, has worked 99.9% of
the time (the 0.1% loss of communications was due to an exceptionally
heavy snowstorm).
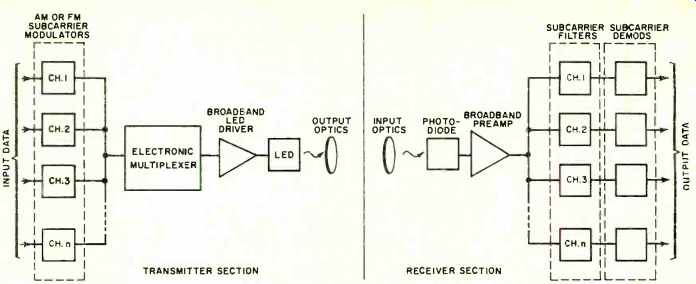
Fig. 2. Block diagram of an "n "channel type frequency-multiplexed
LED transceiver.
A second kind of LED frequency-multiplexing scheme, often called color
multiplexing, uses a separate LED for each channel of information. By
using a selection of LED's that have slightly different spectral outputs,
it is possible to intensity-modulate each LED and combine the outputs
into a single, multi-color beam. A set of photodiodes, each spectrally
matched to one of the LED's, separates the channels at the receiver end
of the communications link.
Color multiplexing eliminates the need for elaborate electronic frequency-multiplexing
circuitry, but the scheme suffers from one big disadvantage: LED's using
silicon or gallium-arsenide have a preference for operating in the 9000-angstrom
range. LED's doped to operate in the 8000 to 6000angstrom (visible red
and yellow) range have substantially lower outputs than the typical infrared
version. Future LED developments may solve this problem; but until that
time arrives, color multiplexing will be restricted to very-short-range
optical coupling applications sending 6 or 8 channels of computer data
across a room, for example.
With a typical digital capacity of 50 megabits per second, LED communicators
show great promise in time-division multiplexing. Since time-multiplexed
LED communicators can handle hundreds of computer data channels simultaneously,
all the major computer firms hope to begin supplying LED links for local
time-sharing traffic. Compared to the voice-grade telephone lines that
carry most time-sharing traffic today, the data capacity of a single LED
link is virtually limitless. LED links will not only make it easier to
connect more teletypewriter terminals to a computer facility, but will
make high-capacity operations, such as interactive graphics, more feasible
than they are at present.
Right now, spatial multiplexing is the least versatile of the three LED
multiplexing schemes. Spatially multiplexed LED communicators use one
LED for each data channel; but unlike the color multiplexing operation,
LED's in the spatial scheme may have the same output wavelengths.
The trick is to keep the light beams separated from one another so that
they fall onto an array of receiver photo diodes in a one-for-one pattern.
This is no small trick, considering LED light is only quasi-coherent and
thus has a tendency to "smear" as it moves through space.
One way to keep the beams from becoming hopelessly scrambled in transit
is to run them through sets of collimating lenses spaced at regular intervals
along the transmission path. A more effective technique is to couple each
LED to the proper photodiode through fiber optics. Since the frequency
and time-division multiplexing schemes do not require any kind of optical
manipulation along the transmission path, spatially multiplexed LED communicators
are pretty much out of the running in most OCL applications.
Their only real promise appears to be in across-the-room types of applications.
Laser diodes, or injection lasers, produce coherent light when forward
biased with a current that exceeds a lasing threshold of about 2 amperes.
Laser diodes can also produce up to 4 watts of output power with efficiencies
on the order of 40%. Unfortunately, the optimum operating temperature
for laser diodes is about 77°K (room temperature is 300°K). Operating
a laser diode at room temperature degrades its performance to a point
where it can barely compete with an inexpensive LED. Laser diodes must
be operated in a pulse mode at room temperature. State-of-the-art laser
diodes have minimum pulse response times in the neighborhood of 0.1 microsecond
and duty factors of less than 1%. Thus, the peak operating frequency
is around 100 kHz. It is possible to achieve higher pulse repetition rates,
but the duty factor then limits the power output to a fraction of a watt.
The only feasible kinds of direct modulation for a laser diode communicator
are those that use pulse-modulation techniques-pulse-frequency or pulse-displacement
modulation, for example. Even then, the extremely low repetition rates
limit the information capacity to one or two voice-grade channels or a
single 50-kilobit-persecond data channel.
In spite of these severe limitations upon their information capacity,
single-channel laser-diode communicators manage to hold their own in the
industrial and military marketplace. The real advantage of laser-diode
communicators is that the coherence and high power outputs make it possible
to reach ranges on the order of 10 miles without resorting to large-aperture
optics. The signal-to-noise ratio for laser-diode communicators is much
greater than that possible with LED versions.
The Santa Barbara Research Center, a subsidiary of Hughes Aircraft, now
markets a laser-diode transceiver designed to carry a single channel of
PFM voice or digital data. The transmitter drives the laser diode with
100-ns pulses that are frequency-modulated around a 6-kHz carrier. Using
only 1-inch lenses and constructed in a binocular arrangement, the system's
peak power output of 2 watts gives these communicators an operating range
of 6 miles.
Compared to conventional gas or solid-state laser sources, semiconductor
light sources are more rugged, smaller, cheaper, and easier to modulate
and require much lower operating voltages. These features make semiconductor
OCL equipment especially suited as portable communicators or short-haul
optical links that require little routine maintenance.
On the negative side of the ledger, the semiconductor sources have much
lower output capabilities than conventional lasers. In outer space where
the line-of-sight range is limitless, OCL's using high-power conventional
laser sources can have operating ranges measured in thousands of miles.
Although OCL's established around conventional laser sources are elaborate
and expensive affairs, their long haul capability justifies their existence.
|