For constant reference while working on repairs, the TV technician’s constant
companion should be a schematic diagram. Certainly the schematic diagram is
one of the most important tools of anyone who works with electronic or electrical
equipment. If you expect to do any TV repair work, or have anything to do with
handling electrical or electronic equipment, you need a working knowledge of
these diagrams. This guide was created with the assumption that you already
have some experience in reading schematics; but this appendix is included to
give some pointers on the specifics of television schematics.
Schematic Diagram Basics
Schematic diagrams are designed to provide a graphic presentation and analysis
of electrical equipment components, and how they are connected. However, the
schematic diagram is not an explanation of the function. Rather, the technician
must be able to interpret the diagram. The schematic diagram should not be
confused with other types of diagrams, such as block diagrams, wiring diagrams
or layout diagrams. Because schematic diagrams show only basic electrical circuit
functions, and not physical characteristics, a diagram often will be exactly
the same for circuits that appear to be different. Conductors and components
in a schematic diagram are arranged in such a way that they do not resemble
their actual physical arrangement. Arranging everything in a schematic diagram
to conform with the actual features of an electronic component could make it
hard for the technician to interpret the circuit function of the components,
which is, after all, the primary purpose of the schematic diagram.
Component names and connections are apparent to anyone who examines the equipment.
Understanding how a component functions requires knowledge of the circuits
being used. This is where the schematic diagram is the most useful: when the
technician is troubleshooting, repairing, and operating the electronic components.
Here are a couple of basic tips on reading general schematic diagrams, if
you aren’t familiar with using them. First of all, straight lines represent
all connecting conductors, despite the type of conductor being used, whether
a huge bus bar or a tiny wire. Several different types of conductors could
be used in the component in question, but in the schematic diagram they will
all be depicted as simple straight lines. Occasionally, a heavier line is used
to depict a heavy ground bus or a metal chassis; but for the most part, all
conductor lines are thin, regular lines.
If some conductors within a given circuit connect, a dot is placed at the
point where the conductor lines cross, or where one conductor line ends as
it reaches another. If conductor lines cross but do not connect, there is no
dot; sometimes a “jumper” is drawn to show that one conductor jumps over the
other instead of connecting to it.
The Television Schematic
The schematic diagram of a typical television receiver is rather complex.
The television sound system is identical to that of FM radio, while the picture
system is more like an AM system; except, of course, that pictures rather than
sound are broadcast.
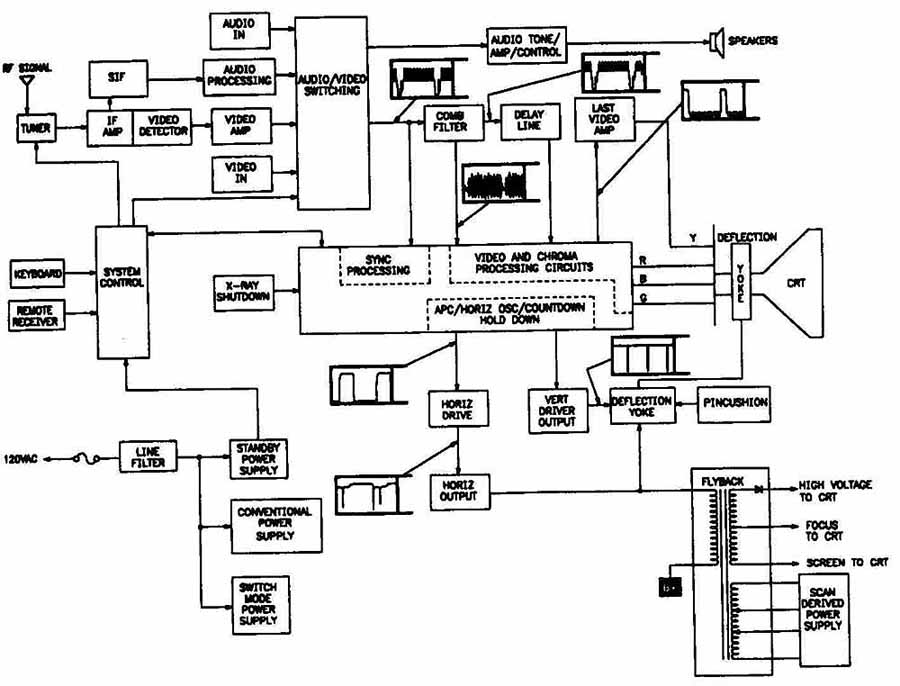
FIG. 1. Block diagram of a color television receive, showing the black-and-white
stages.
The schematic diagram for a typical television receiver is rather large and
complex, so we shall first examine the overall block diagram before looking
at specific components within the schematic diagram. (See FIG. 1.)
The Receiver Block Diagram
The location of any circuit within the actual television set is important
in interpreting the overall schematic diagram. For this reason, we will first
review the TV circuits.
Color television, of course, dominates the TV broadcast industry, but many
black-and-white sets are still in use. The only difference between a color
receiver block diagram and one for a black-and-white set is that a separate
sound detector isn’t used in the black-and-white set. The video detector is
used for both functions, and the sound IF signal moves from the video detector
to the sound IF amplifier.
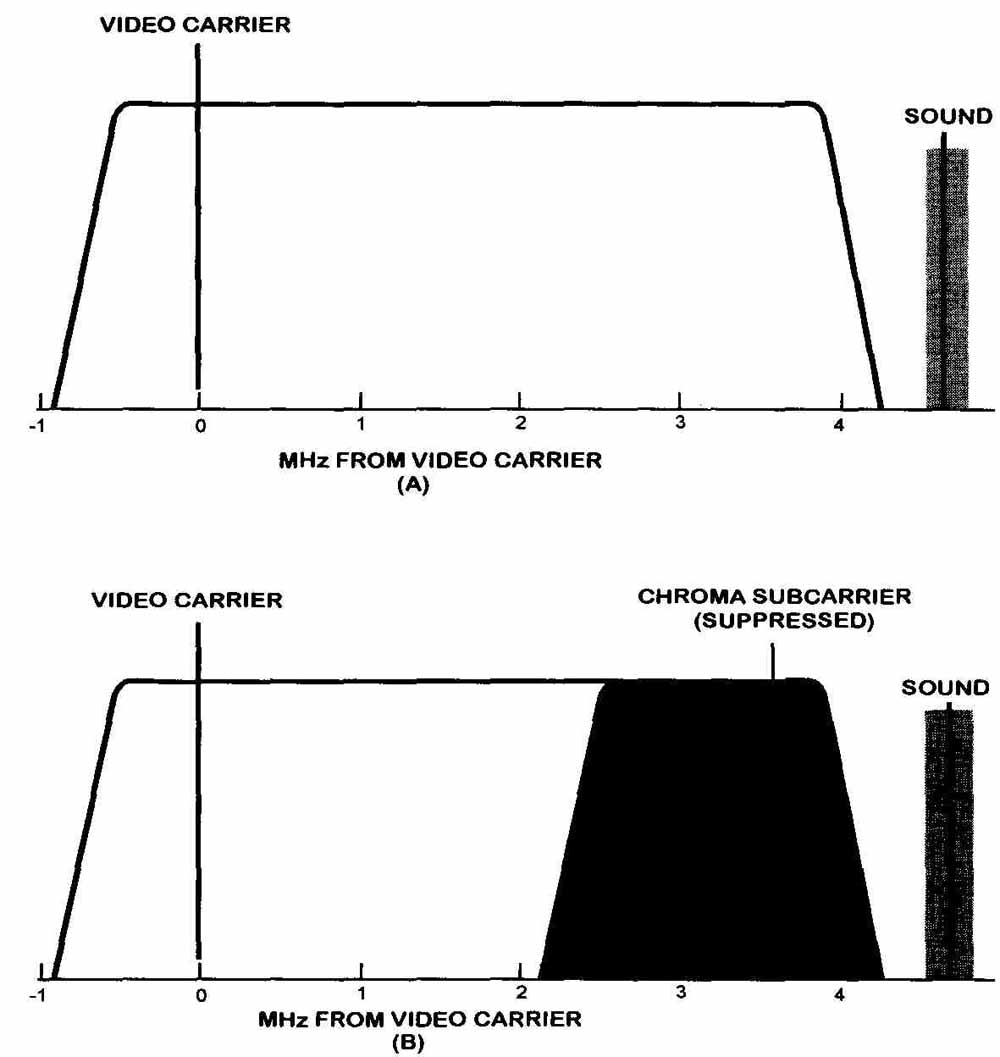
FIG. 2. Black-and-white (A) and color (B) television signals.
The antenna signal enters through the tuner or front end, the section that
contains the oscillator, the mixer and the RF amplifier. Included in the signal
are the frequencies of the black-and-white and color signals ( FIG. 2). Please
note that there are two separate carriers, one each for sound and picture.
In addition, the bandwidth of the signal is almost 6 MHz: the frequency space
set aside for each television channel. This bandwidth also includes the sound
and picture side bands. Back in FIG. 1, the frequency components are contained
in the tuner and video IF amplifier sections, after which the amplified signals
are split into the sound and video detectors.
The mixer output contains the video IF and sound IF signals; the sound signal
is 4.5 MHz lower than the video signal’s frequency. Both the sound and video
IF signals are amplified by the video IF amplifier, which also applies them
to the video and sound detectors. These signals mix (hetero dyne) in the sound
detector to produce a third signal with a carrier frequency of 4.5 MHz (the
difference between the carrier frequencies of the two IF signals). The carrier
is frequency modulated by the audio signal and, to an extent, amplitude modulated
by the video signal. The limiting action of the amplifier stage removes the
amplitude modulation. The 4.5 MHz sound IF signal is amplified in the sound
IF amplifier, and demodulated in the sound demodulator, creating an AF signal.
The AF signal is then amplified in the AF amplifier and sent to the loudspeaker.
At least one IC is used in the sound section of most receivers.
The video IF amplifier output goes through the video detector, where the video
elements of the signal are separated. The video elements consist of information
for a black-and-white picture (luminance), color (chrominance) and synchronization
between picture and color (sync).
In FIG. 1, you can see the destinations of the signals used for black-and-white
reception. The video signal moves from the video amplifier to the grid of the
picture tube. The sync elements move to the sync section, where they are used
to control the vertical and horizontal sweep oscillators. The sync elements
also supply pulses for the high-voltage power supply and occasionally part
of the low-voltage supply.
The other color sections are shown in FIG. 3. You can see that the luminance
pail of the signal encounters a delay line in its path. The delay line holds
the luminance signal just long enough to allow the other color signals to “catch
up” in their respective sections; therefore, all color signals reach the picture
tube at the same moment.
In the meantime, the chrominance elements leave the video amplifier to be
filtered and amplified in the chroma bandpass amplifier. From there, they move
through the chrominance demodulator, then to the picture tube. The two chrominance
signals, R-Y and B-Y, are separated by the demodulation process and then combined
to produce the G-Y signal. The red (R-Y), blue (B-Y) and green (G-Y) signals
(known as color or difference signals) are then applied to the picture tube,
or matrixed to produce red (R), green (G) and blue (B).
The color burst and sync signals, derived from chrominance information, are
used to put the color video elements in sync, by synchronizing the subcarriers
in the chrominance demodulator. These signals move from the chroma amplifier
to the burst amplifier, which separates them. The color killer switches off
the chrominance amplifier if there are no color signals.
As shown in FIG. 4, the tuner stage selects one channel from all of the signals
received by the antenna. Each channel consists of an RF frequency containing
two carriers: the FM sound carrier and the AM video carrier. The tuner circuit
adjusts the lever of the RF signal and converts it into an IF signal. So, the
tuner circuit has one input, the RF frequency, and one out put, the IF signal,
though there are two carriers in the RF frequency. See Section 9 for tips on
troubleshooting tuners.
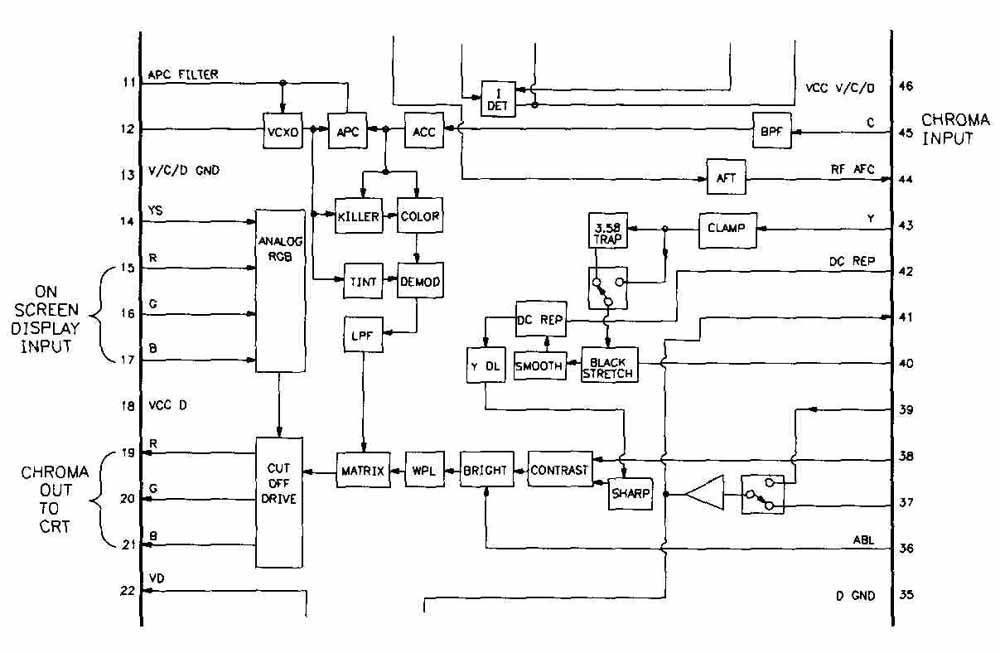
FIG. 3. A color TV color sections.
The Tuner Stage
Each of the television stations in a geographic area transmits on a specific
frequency. To select one signal and have all of the other stages in the chassis
recognize the selected signal without having to constantly retune, televisions
use a tuning method called heterodyning.
In heterodyning, when you select a channel, the RF amplifier is tuned to that
frequency. The RF amplifier amplifies the signal and outputs it to the mixer
stage. Then, the oscillator outputs its signal reference to the mixer. These
two input signals are mixed so that the output signal from the mixer is the
difference between the two signals, or the intermediate frequency (IF). All
of the later stages in the chassis are permanently tuned to the IF so that
they do not have to be retuned each time you select a new channel.
There are two types of tuner circuits. A “discrete” tuner is composed of individual
components that are part of the main board. If a discrete tuner is not working
correctly, you can test the components, locate the one that is faulty, and
repair it. A “modular” tuner is a replaceable unit that is easier to replace
than try to repair when it is faulty. FIG. 5 shows a block diagram for a “generic”
tuner circuit.
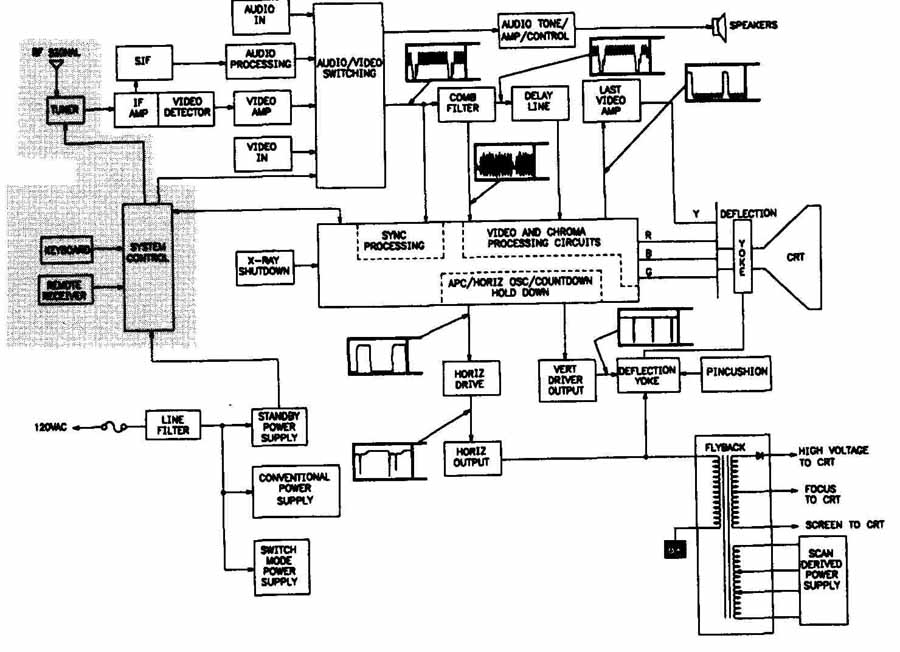
FIG. 4. The tuner stage (shaded).
Manufacturers have developed several types of tuners, including varactor (variable
capacitance diode) and frequency synthesis tuners. However, all tuners, including
the older mechanical tuners and hybrid tuners, have three main tuner stages:
RF amplifier, oscillator and mixer.
The RF amplifier provides frequency selectivity, improves the signal-to-noise
ratio, and prevents the radiation from the oscillator from leaking back to
the antenna. The RF amplifier stage, shown in FIG. 6, selects one RF signal
from all of the signals being received by the antenna, amplifies it, and outputs
it to the mixer. The AGC (automatic gain control) is connected to the RF amplifier
and controls the gain of the amplifier by offsetting variations in the carrier
signals. The RF amplifier can be a transistor or a FET (field effect transistor).
The television’s antenna is part of the RF amplifier circuit. The RF amplifier
blocks the signal from the oscillator from leaking back to the antenna. Signal
leaking can cause picture interference.
The oscillator stage, like the one shown in FIG. 7, outputs an unmodulated
reference signal to the mixer that tracks the desired RF signal at a fixed,
offset frequency. The frequency of the signal is manufacturer dependent.
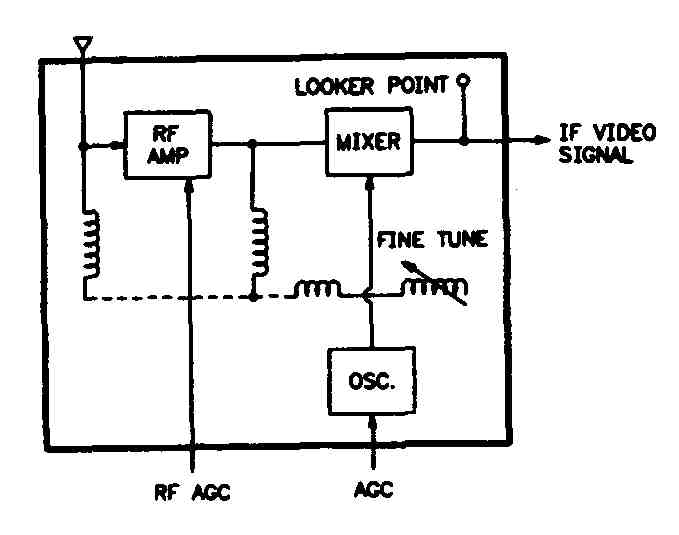
FIG. 5. A generic tuner circuit.
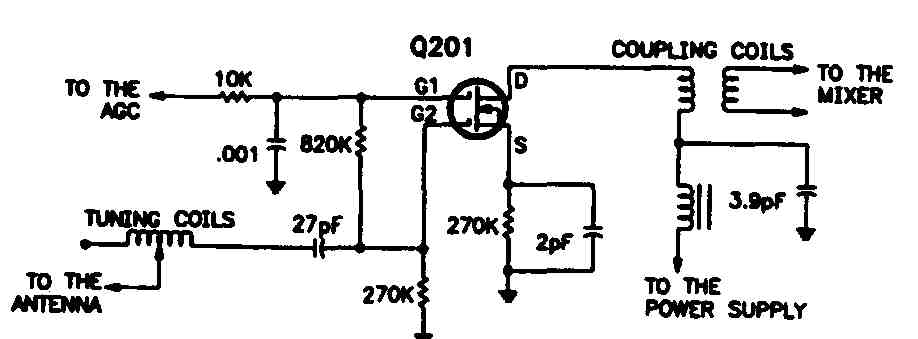
FIG. 6. An RF amplifier.
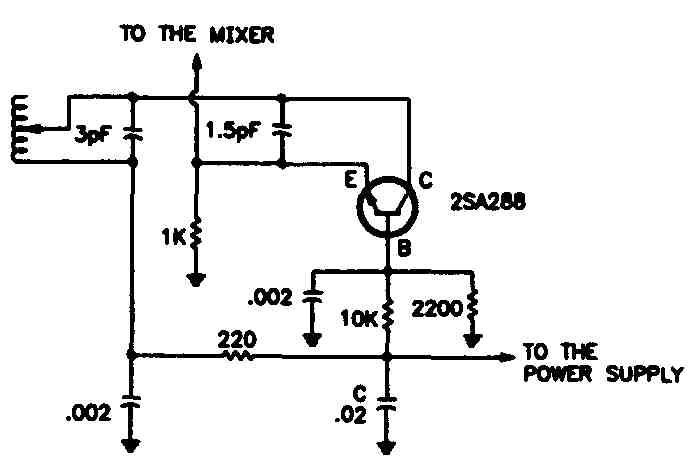
FIG. 7. The oscillator stage.
Also, tuners have an AFT (automatic fine tuning) circuit connected to the
oscillator. The AFT shifts the oscillator frequency as needed to keep the signal
in phase with the selected channel frequency. Older televisions have manual
fine tuning controls that keep the signal in phase with the channel frequency.
The mixer stage, like the one in FIG. 8, inputs the RF and oscillator signals,
mixes them, strips out the extraneous signals from the original RF and oscillator
signals, and produces the IF signal. The conversion must be performed without
distorting the incoming signal. Mixers are sometimes called frequency converters,
frequency translators or heterodyne detectors.
The output from the mixer is an IF signal, as shown in FIG. 9. The frequencies
represented in the signal are constant so that the signal can be used in later
stages in the chassis:
39.75 MHz — The adjacent channel’s video carrier.
41.25 MHz — Audio carrier.
41.67 to 42.69 MHz Color carrier.
45.75 MHz — Video carrier.
47.25 MHz — Adjacent channel’s audio carrier.
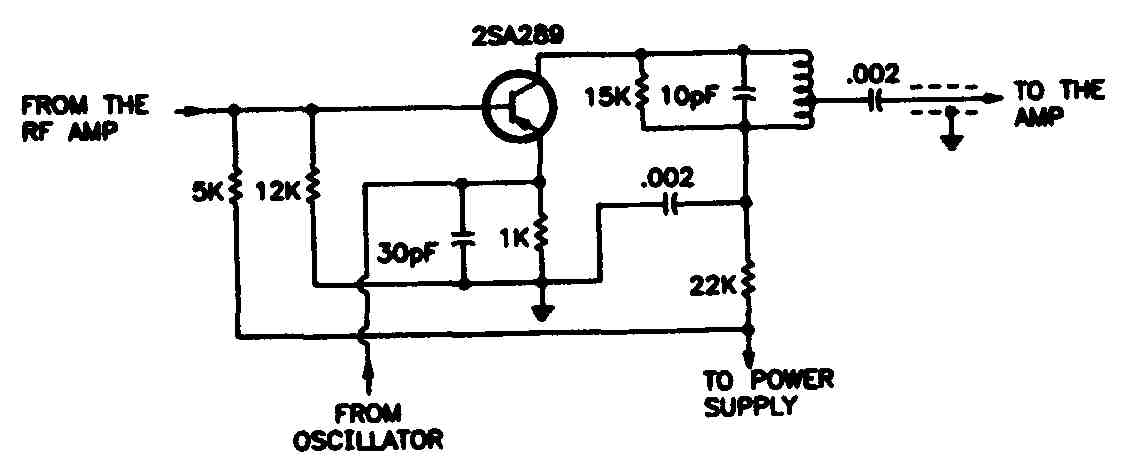
FIG. 8. The mixer stage.
Listed here are only three of the types of tuners you will see. The design
of most tuners is dependent on the individual manufacturers. A varactor tuner
is a diode that acts like a variable capacitor when the diode is biased by
a variable voltage supply. The capacitance can be changed over a large range
of frequencies by inputting a control voltage from the regulated low-voltage
power supply. When a channel is selected, a pre-selected voltage for that channel
is applied to the diode. This causes the diode to align with the selected frequency.
When you fine-tune the frequency, the applied voltage is adjusted using an
AFT correction voltage until the diode is completely aligned with the frequency.
A reactance tuner consists of coils and a capacitor. Each coil is assigned
to a tuner frequency. The coils are connected with a multiple contact switch
or a series of diodes used as switches. When a new channel is selected, the
switch contacts another coil, and the channel changes.
A frequency synthesis (FS) tuner, also known as digital tuning or quartz tuning,
provides up to 127 channels and all of the UHF frequency band. The ES circuit
is an IC that contains additional stages such as a phase-lock loop (PLL), step
generator, a frequency band switch decoder, an AFT, digital sync, and a presence
decoder. Refer to the schematic for details about the FS tuner.
Phase-Locked Loop (PLL) Synthesizer
System
The phase-locked loop (PLL) is a system through which a frequency signal can
be frequency stabilized through the control of a standard reference oscillator.
The operation of a PLL system is depicted in FIG. 1O.
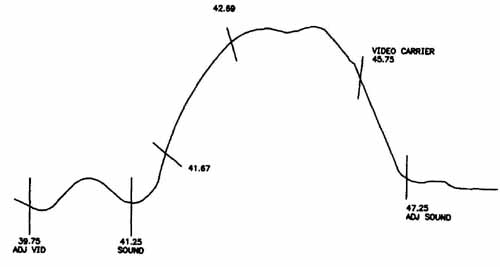
FIG. 9. An IF signal waveform.
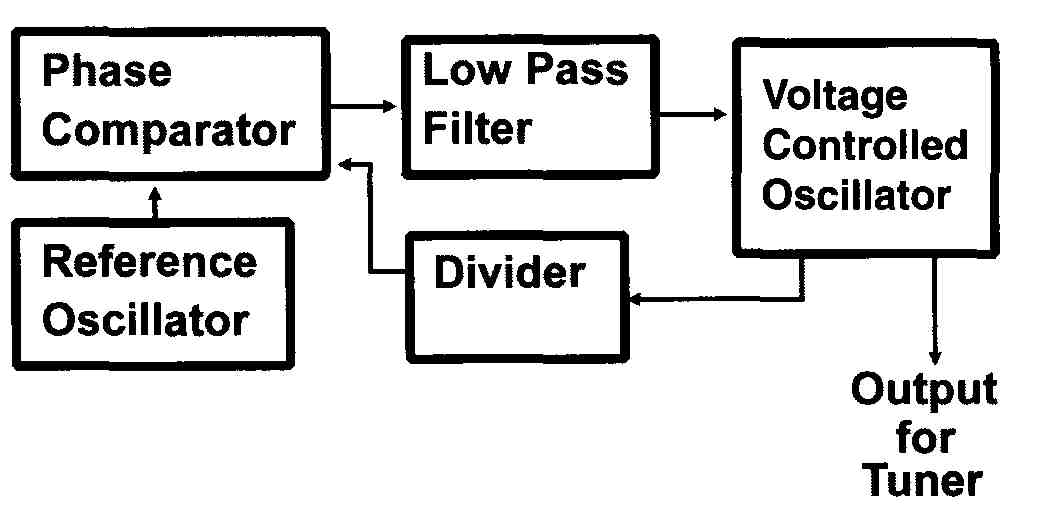
FIG. 10. A phase-locked loop (PLL) synthesizer system.
The varactor in the voltage-controlled oscillator (VCO) circuit varies the
oscillator frequency according to a DC voltage being applied to the varactor.
Usually, the reference oscillator is a fixed-frequency, crystal-controlled
circuit. In FIG. 10, the reference oscillator frequency is relatively low,
so its stability is ensured. VCO and reference oscillator outputs are applied
to a phase comparator. The VCO signal is passed through a divider that reduces
its frequency so it comes close to matching the reference oscillator in value
(the VCO frequency is higher than that of the reference oscillator).
The VCO and reference signal phases and frequencies are compared in the comparator.
If the signals are different in any way, a DC (or difference) voltage develops.
The difference voltage moves back into the VCO to correct the oscillator frequency,
so that it matches that of the reference signal.
In FIG. 10, the reference setting fixes the received oscillator frequency
and channel, since programmed dividers can operate at any integral multiple
of the oscillator frequency.
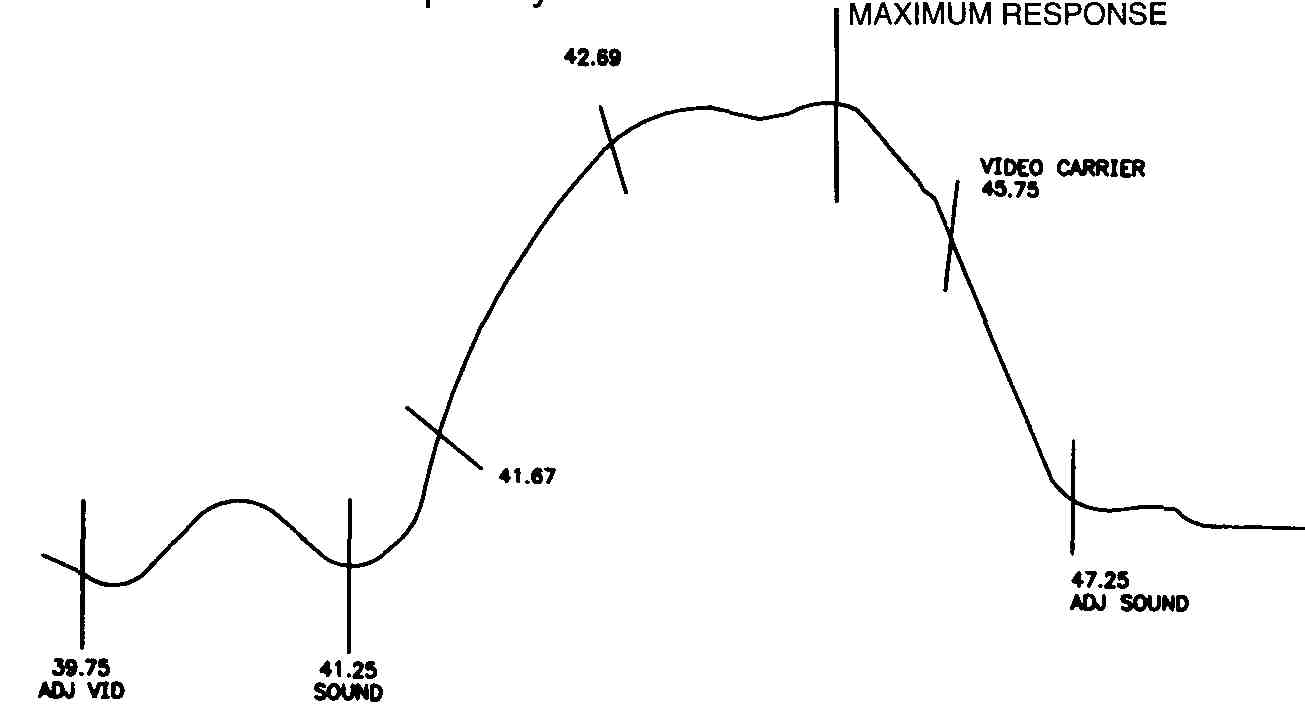
FIG. 11. An IF signal waveform with maximum response curve indicated.
Video
IF amplifiers used for television video are similar to those used in radios.
The television IF is designed to amplify a wide band of frequencies (almost
6 MHz) to include the carriers and side bands shown in FIG. 2. The video IF
amplifier must have a frequency response that maintains the ratio of the video
and sound IF amplitudes (see FIG. 11).
Most television receivers use ICs in the IF amplifier, so ICs are indicated
in the amplifier section in a schematic diagram. FIG. 12 shows a typical IF
section. Note that one IC (shown in three parts, IC1A, IC1B and IC1C) is used
for the IF and detector sections. The tuned circuits are external to them,
and the IC contains the active components, such as the transistors. IF alignment
is accomplished through the IF transformers, which is why they are kept physically
accessible. FIG. 12 also shows the video detector and the first video amplifier
circuit.
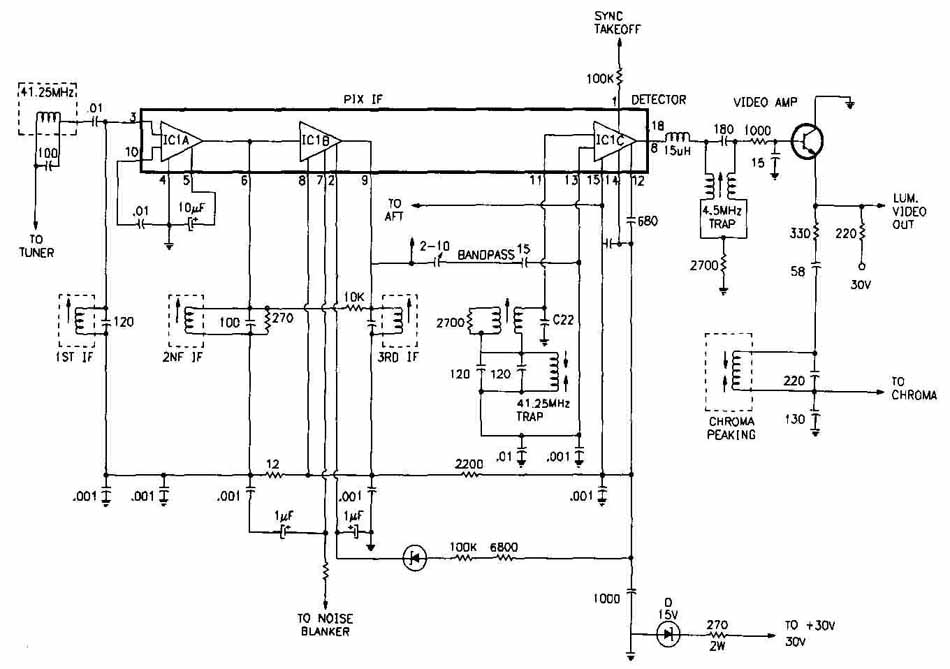
FIG. 12. An IF amplifier section, connected to an AGC.
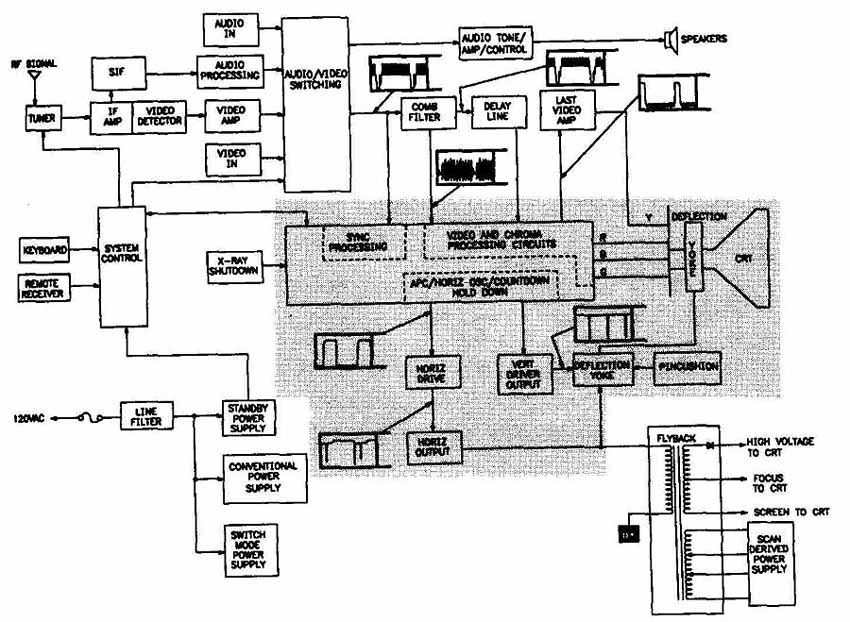
FIG. 13. The television picture sync and deflector circuits (shaded).
The coupling transformer between IC3 and the video amplifier acts as a trap
to eliminate the 4.5 MHz sound IF signal from the video and sync composite
signals. The sound IF signal is from the output of IC2, as indicated in FIG.
12. There is a separate detector for sound in color TV receivers. The sound
detector separates the sound IF signal from the video IF signal. The resulting
signal is a mix of the high-frequency sound IF (41.25 MHz) and video IF signals
(45.75 MHz). Its frequency is the difference between them.
When the video signal is retrieved from the IF signal, it is put through several
stages (at least two) of amplification. The video amplifier supplies signals
to three other receiver sections, consisting of the picture tube (through matrices),
the chrominance amplifier and the picture sync section. The luminance or Y
signal goes to the picture tube. The Y signal contains all the signal components
for monochrome or black-and-white video. The luminance signal moves through
the picture tube matrixing circuit, where it is mixed with chrominance to produce
picture tube grid and cathode signals.
Sync and Deflection
Sync pulses in the video signal are used to keep the vertical and horizontal
deflection signals in sync with the received signal. Sync pulses are divided
into vertical and horizontal pulses, separate from the video signal. Sync circuits
compare sample pulses from the receiver’s deflection circuits with the received
pulses, and synchronize the frequency and phase of the received picture.
FIG. 13 shows a block diagram of the picture sync and deflection circuits.
The separated vertical sync pulses move directly to the vertical deflection
section, where they control the vertical oscillator frequency. When applied
to the automatic frequency control (AFC) circuit, the horizontal sync pulses
are compared to the pulses from the horizontal deflection circuit. Differences
between the two results in a correction voltage being fed from the AFC circuit
to the oscillator. The deflection signal sample used for comparison in the
AFC is usually taken from the horizontal deflection section output stage.
FIG. 14 shows a vertical deflection circuit. The sync pulses trigger each
cycle and keep the sweep in sync with the picture, when applied to the oscillator
base circuit. The oscillator feeds a vertical pulse to the vertical output
IC. The IC processes and compares the vertical signals in order to maintain
a proper vertical output. The output moves to the vertical deflection yoke
windings to ‘sweep’ the picture tube beam.
FIG. 15 shows a horizontal deflection circuit. Here the oscillator is synchronized
by a DC voltage from the AFC circuit. This voltage corrects deviations of the
oscillator frequency and phase. In the output stage, a power type transistor
is used, which produces horizontal blanking to cut off the picture tube beam
during retrace, current for pincushion magnets, heater current for the picture
tube and pulses for the high-voltage power supply. The transistor can also
be a source for a low-voltage power supply.
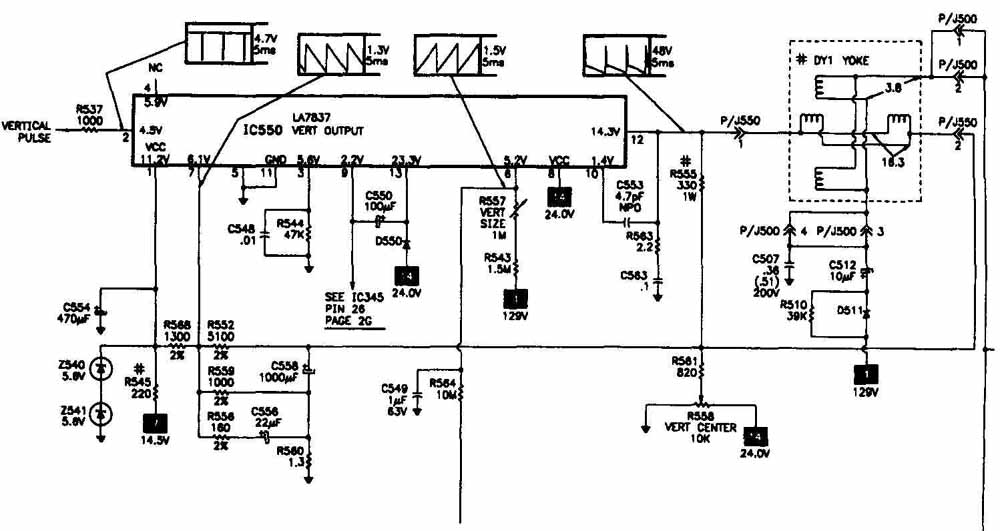
FIG. 14. Vertical deflection circuit.
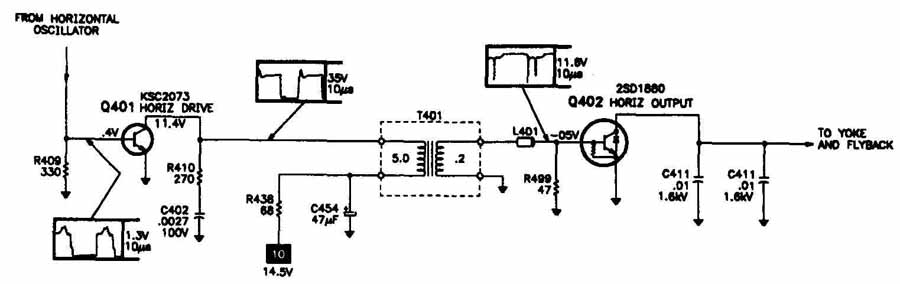
FIG. 15. Horizontal deflection circuit.
Sound
FIG. 16 shows a block diagram of a sound section. In the video IF signal,
the sound and video carriers are 4.5 MHz apart. The IF signal, which contains
both signals, is fed to a separate detector in which the two signals are mixed,
or heterodyned. The resulting difference heterodyne, based at 4.5 MHz, is used
as the sound IF signal. To keep the FM sound modulation dominant in the 4.5
MHz sound IF signal, the high-frequency sound IF signal is maintained at a
lower level than the video IF signal. Then, the 4.5 MHz IF signal is amplified
and moved to the FM demodulator. The AF signal that results is then amplified
in a regular amplifier.
The AF section is either all or partly included in an IC in nearly all television
receivers. FIG. 16 shows an AF division into two ICs. FIG. 17, on the other
hand, shows a circuit in which all AF components and the sound IF amplifier
are included in one IC. The three main functions accomplished in the signal
IC are illustrated in FIG. 17(A) and (B). Only the sound IF amplifier and demodulator
are included in the IC in FIG. 18. Discrete components are used in the AF stages
that follow.
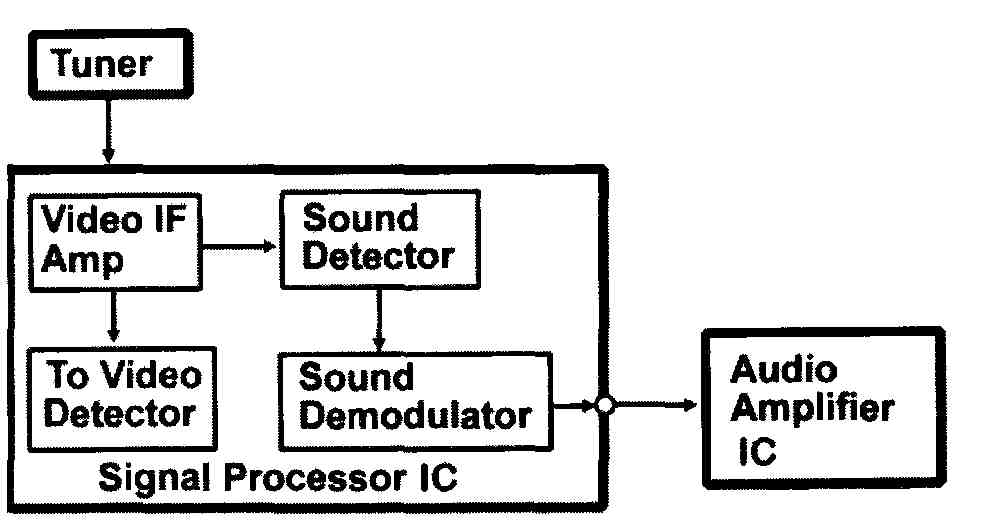
FIG. 16. Television sound system.
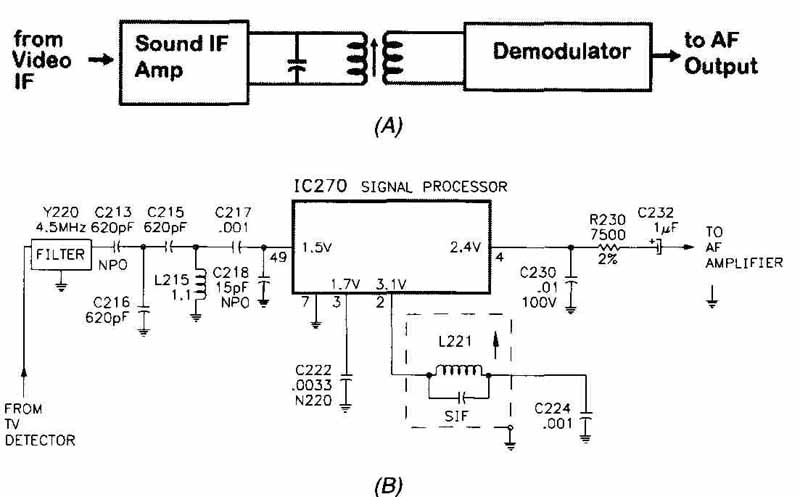
FIG. 17. An IC sound section showing (A) major portions of the IC, and (B)
an example circuit using ICs.
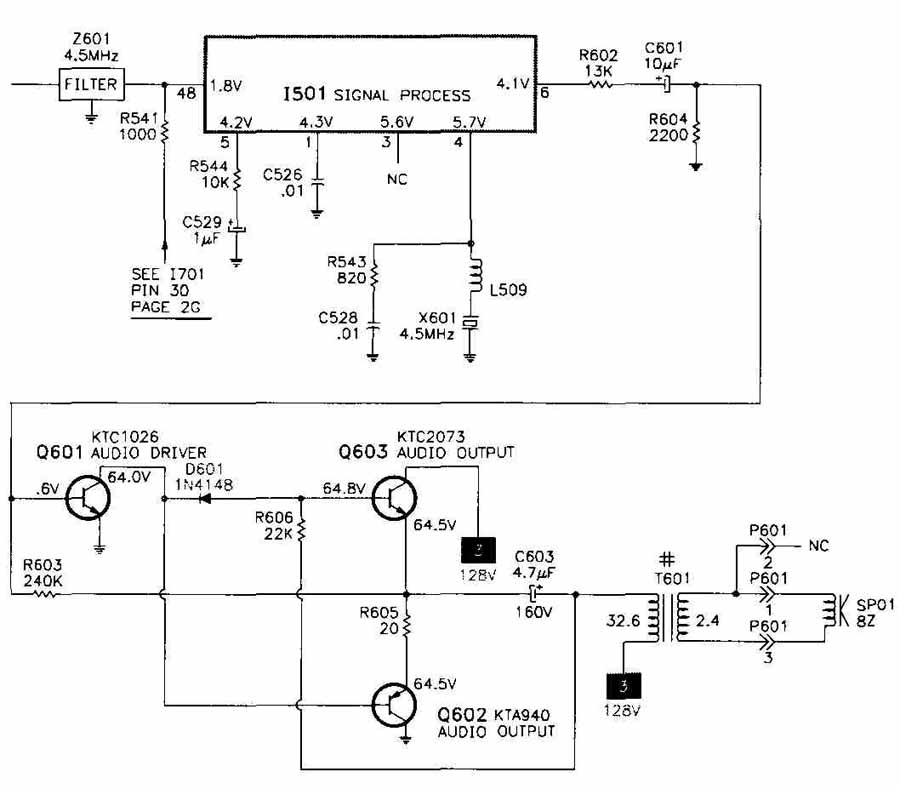
FIG. 18. In this sound section, only the sound IF amplifier and demodulator
are included in the IC.
Power Supplies
Low-voltage and high-voltage are the two power supplies used in a TV receiver.
Low-voltage supplies provide current to the screen grids of the picture tube,
to transistors, and to other devices requiring voltage in the 5- to 200-V range.
The high-voltage power supply, 20 to 30 kV, is used for the anode and for the
focus anode of the picture tube, 5 to 15 kV. All high- voltage (HV) supplies,
and a few low-voltage ( LV) supplies, get their input from the horizontal output
stage. Filtering is much simpler than with 60 Hz supplies because this current
is at a frequency of 15,734 Hz. Some kind of 60 Hz supply must always be used,
however, because current is needed to provide energy for the 15 kHz supplies,
to operate the horizontal deflection section.
FIG. 19 shows the configuration of the 60 Hz LV power supply. In this example,
a bridge rectifier supplies a 130 V regulator. These voltages are used to provide
the necessary power for the horizontal deflection circuit. FIG. 20 shows the
other type of LV supply, operating from the horizontal output circuit. In this
case, the AC voltages are taken from the transformer at the output of the horizontal
output transformer. Note that a Zener diode regulator is used for the 12 V
output.
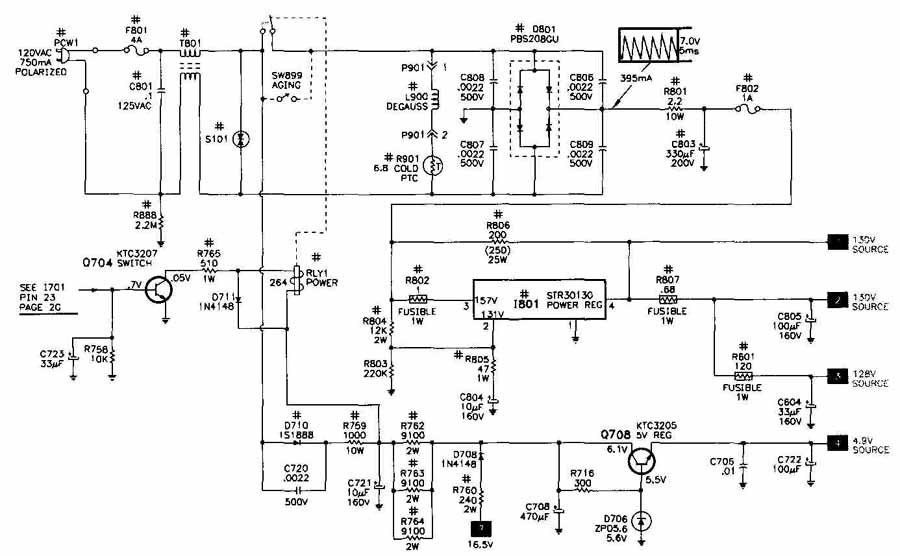
FIG. 19. The use of a 60 Hz low-voltage power supply.
High-voltage power supplies get their power from the horizontal output stage.
A winding on the horizontal output transformer, or an extension of another,
provides high-voltage pulses that are rectified or passed into a voltage multiplier.
This in turn steps up the voltage while rectifying the signal. A typical high-voltage
power supply circuit is shown in FIG. 21. Focus and screen voltages, needed
for the picture tube, are also obtained from this supply.
Chroma Amplifier
The chroma, or chrominance amplifier (also called a bandpass amplifier) receives
the signal from the video amplifier. This amplifier separates the chrominance
side bands from the rest of the video signal. FIG. 2shows the frequency arrangement
of the side band signals. The chroma side bands are part of the video signal,
forming a modulated signal that must be de modulated in order to receive the
chroma signals.
In FIG. 2(B), note that the chroma side bands are in the upper part of the
video IF spectrum, from 3 MHz and up. So these side bands are de modulated
and used properly, they are separated from the luminance video (Y signal) occupying
the range under 3 MHz. The chroma amplifiers job is to handle this separation.
The amplifier circuit itself is not much different from the video amplifier,
but its constants limit response to the 1 MHz between 3 and 4 MHz.
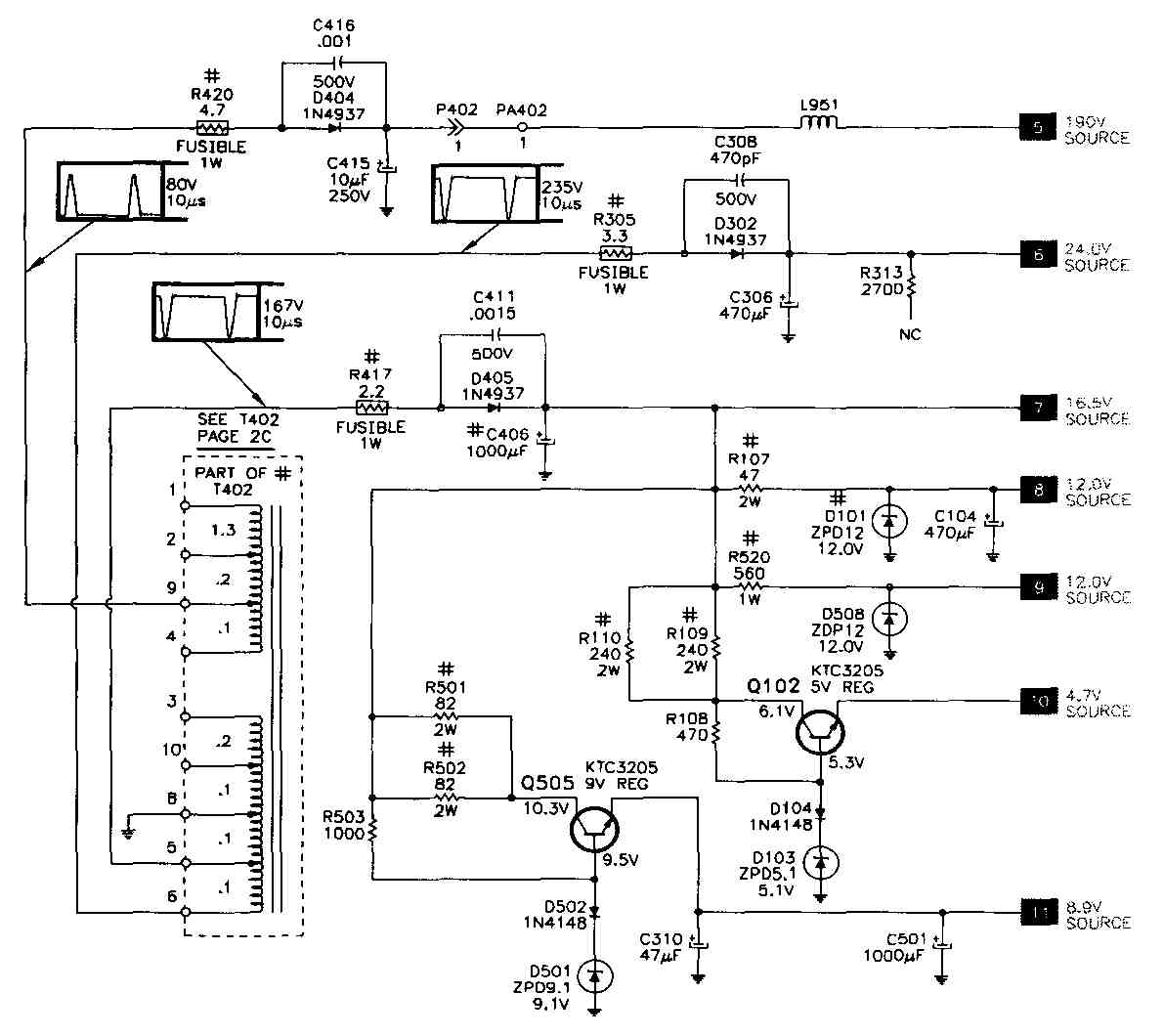
FIG. 20. Here the power supply receives input from horizontal deflection.
Color Sync
Demodulating the chrominance side bands requires the use of subcarriers to
produce color signals that can be used at the picture tube. The subcarrier
signal phases must be in sync in relation to a burst signal along each horizontal
pulse. The color sync portion of the television receiver separates the color
burst signals from the video signal, creates its own subcarrier signal and
attaches it to the burst signal, and applies the resulting signal to the demodulator.
When the original signal is produced in the television studio, the camera
captures the color and sends the signal to a modulator. The modulator circuit
combines the three color signals into one color signal. This circuit also produces
a color sync signal. The color signal is then called a color modulated signal.
The color sync (color burst) signal is an unmodulated 3.58 MHz carrier. See
Section 5 for more details.
A television’s color processing circuits recreate a color picture and produce
the color picture on the screen. Essentially any color can be created from
the primary colors red, green and blue (color difference signals R-Y, G-Y and
B-Y).
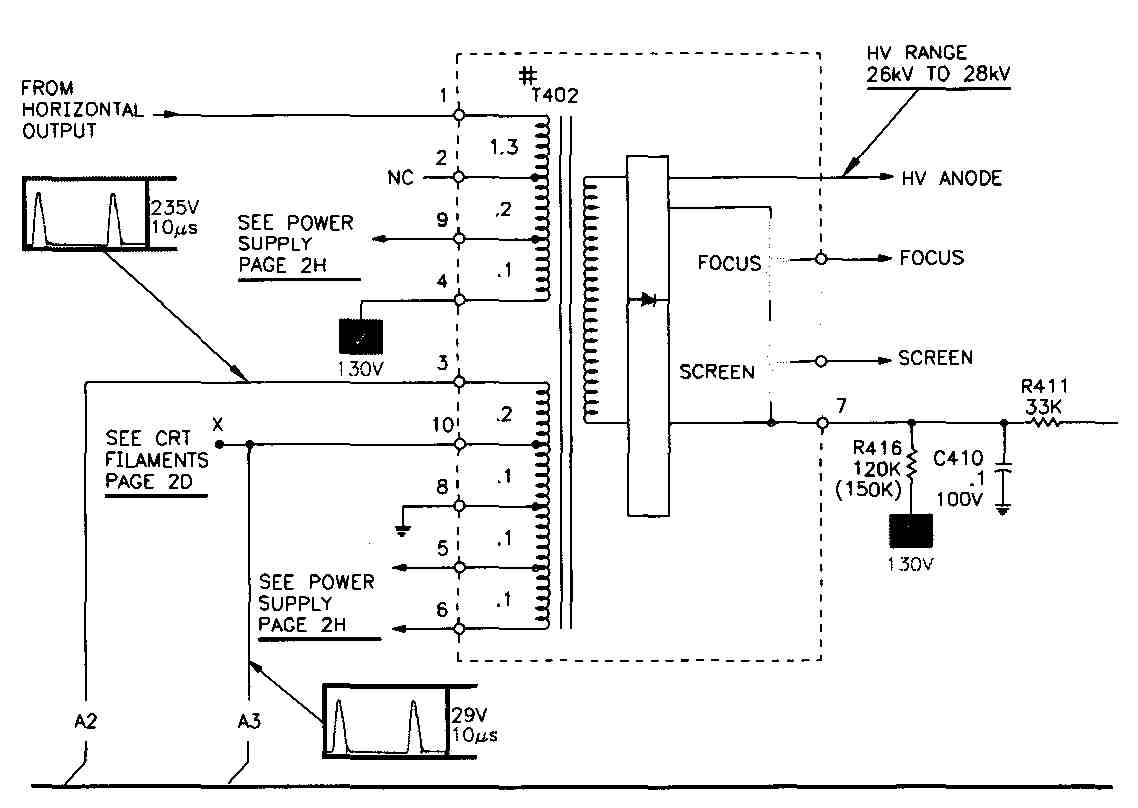
FIG. 21 . A high-voltage power supply circuit.
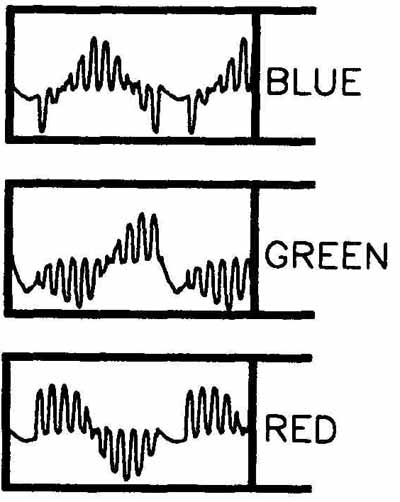
FIG. 22. Red (R), green (G) and blue (B) waveforms.
When the signal is received, the color sync signal turns on the 3.58 MHz oscillator
and synchronizes it with the signal received from the station. The signal from
the oscillator is output to the color killer, which turns on the color IF amplifiers
so that the signal can be amplified. After amplification, the signal is passed
to the color demodulator circuits. In the demodulator circuits the original
red, green and blue signals (RY, G-Y and B-Y) are separated and sent to the
red, green and blue color amplifiers. The color amplifiers combine the three
color signals with the black-and-white luminance information, then pass the
signals on to the three electron guns which pro- duce the color picture on
the screen.
When these circuits malfunction, the result can be no color, color that is
too intense, loss of one color, one or all wrong colors, or loss of color synchronization.
For example, if the demodulator circuits fail, you might see no color, an incorrect
tint or intensity, or intermittent color, tint or intensity. FIG. 22 shows
the red, green and blue color waveforms to expect when testing the color circuits.
(The waveforms are of a color bar pattern.)
Automatic kine bias (AKB) is circuitry used to adjust the beam current of
each electron gun individually. This circuitry is intended to prolong the life
of the electron guns and give optimum color setup. The setup is done only once
in the lifetime of the CRT, or until a malfunction occurs within this circuit.
The AKB IC samples the vertical blanking pulse for timing, and sources a small
bias current to the cathode drivers to draw beam current individually on the
electron guns. The AKB IC senses and adjusts with every vertical blanking pulse.
For an optimum color screen setup, this is critical but relatively easy to
set for most standard chassis.
Picture Tube Application
The color (difference) and Y signals must be combined and fed to the picture
tube when they become available. Most picture tubes have three grids, each
with a corresponding cathode, for the red, green and blue signals. The color
signals are combined with the Y signal to cancel out Y and release R, G and
B.
FIG. 23. Three methods for applying color signals to a picture tube. (A)
shows the color circuits matrixed before the tube, (B) shows the color circuits
at the grids, with the V signal at the cathode, and (C) shows the color signals
being applied by the demodulator to the cathodes.
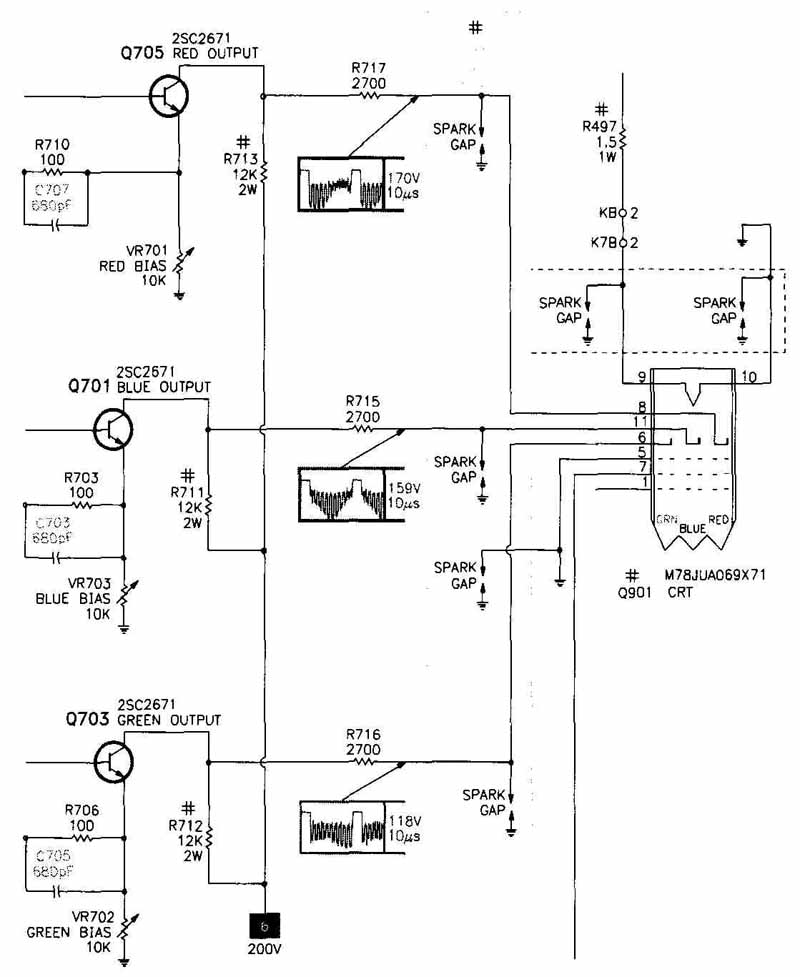
FIG. 24. Color amplifier circuit, showing brightness control and color drive
controls.
FIG. 23 shows some arrangements for combining or applying color signals to
the picture tube. The three color signals are combined in matrices with the
Y signal to derive R, B and G, as shown in FIG. 23(A). These signals are then
applied to their respective grids. The DC-biased cathodes are grounded to the
signals, and the color signals are applied to the grids, as shown in FIG. 23(B).
A -Y signal is applied to the cathodes, which causes the Y signal to add to
the color-difference signals. As a result, one of the three signals, red, green
and blue, is applied between the grid and the cathode of each gun in the tube.
Figure G-23(C) shows that the color signals are applied to the cathodes, and
the grids are at signal ground; the opposite of what is shown in (A).
FIG. 24 shows a circuit for the color amplifier portion of a receiver, indicating
the location of a brightness control and the color drive controls used to adjust
the amplitudes of the three color signals. An arrangement for adjusting the
levels of the screen grid voltages of the picture tube is also shown.
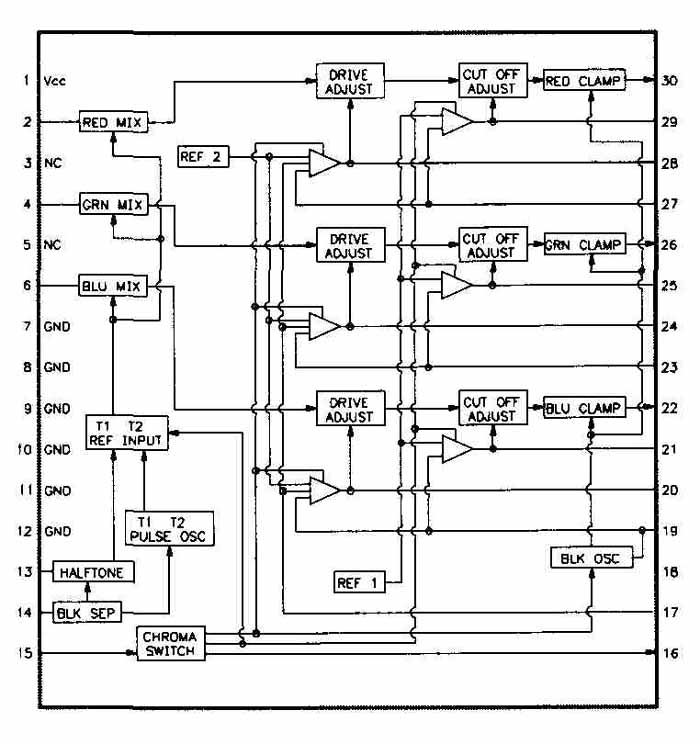
FIG. 25. Circuit using one IC for the color demodulators.
ICs
The receiver color sync section is often referred to as the chroma processor.
Generally, some or all of the sync section is included in one or more ICs.
FIG. 25 shows a circuit using one IC for the demodulators and the following
color amplifiers. The demodulators are supplied by a voltage regulator that
is also in the IC.
Additional Circuits
A television is too complex to require the examination of all of its circuits
within a schematic diagram. At any rate, such a thorough examination is unnecessary
when considering the actual function of the television set. However, let’s
look at the block diagram of a typical receiver to examine automatic control
circuits.
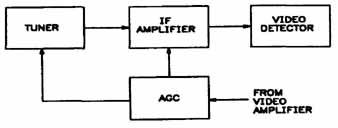
FIG. 26. An AGC system.
Automatic gain control (AGC) reduces receiver RF and IF gain when strong signals
are received, so that they do not overload the amplifiers. FIG. 26 shows a
block diagram of an AGC system. The sync pulse level alone is constant enough
to be used as a measure of signal strength, since the video modulation level
can vary widely during a broadcast. The sync pulse level is determined by separating
the sync pulses at the gated AGC amplifier block. The clamping circuit forms
the pulses into a DC voltage with a value of the received signal level, which
is used as bias on the tuner and IF amplifier. The voltage polarity decreases
the gain of the stages as signal level in creases, so that the signal strength
at the IF amplifier output remains stable.
Automatic find tuning (AFT) is used to keep a television receiver properly
tuned on a station. An AFT circuit is shown in FIG. 27. An IF signal from the
video IF amplifier is fed to a discriminator tuned to the video inter mediate
frequency (45.75 MHz). If video IF signal is at its ideal frequency of 45.75
MHz, where it should be, then the discriminator DC output voltage is zero and
no adjustments are made to the tuner oscillator. If the tuner oscillator is
off its proper frequency, the video IF signal will also be wrong; the result
being that the discriminator will release a DC error signal of a polarity dependent
on the tuner oscillator frequency. The DC error voltage is applied to the varactor,
which changes the capacitance applied to the tuner oscillator, and returns
the oscillator frequency to its correct value.
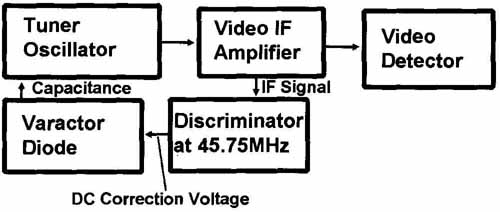
FIG. 27. An AFT system. |