When FM stereo transmission was introduced in the 1960s, millions of
monophonic FM receivers were in use. Whenever anything new is introduced
into a mass market, it is desirable not to make existing equipment obsolete
and to maintain compatibility with existing equipment. Otherwise, buyer
resistance will be met and the new system may not gain acceptance. The
new stereo system had to be backwards compatible with mono FM. The new
stereo transmissions had to be received by existing mono receivers as
monophonic audio, much like color TV transmissions were received as black
and white on the then millions of black-and-white TV sets still in use.
Therefore, a stereo audio format was necessary, similar in principle
to color TV transmission, whereby the main audio channel was transmitted
as the sum of left and right (L+R) audio channels, as the main video channel
transmitted the luminance (brightness components) portion of the TV picture.
The color is transmitted not as color in itself but only as the information
about how it differs from white or neutral grays. Therefore, a black-and-white
receiver would simply reject the color signal and use only the luminance
component to display a black-and-white image. Similarly, the main audio
channel in FM stereo is the total of left and right (L+R) audio signal
components. In addition, a signal is transmitted that is the difference
of the channels (L-R). By adding the signals, the right channel cancels
and the left channel alone appears. By subtracting the (L+R) and (L-R)
signals, the left channel cancels and the right channel similarly is
now obtained. A mono receiver would reject the (L-R) component and reproduce
only the (L+R) component as a mono signal.
The trouble with this idea is that the sum and difference channels (L+R)
and (L-R) both are audio, occupying the baseband of 20-15000 Hz. Using
analog methods, in those pre-digital days, there was no way to separate
the channels as is. So instead, the difference (L-R) component was superimposed
on a subcarrier in the ultrasonic range, where it would be inaudible.
A pilot carrier must also be transmitted to assist in demodulating this
subcarrier back to audio. The stereo receiver would have to extract this
subcarrier and pilot signal and process them to recover the (L-R) difference
signal as audio, then perform the adding and subtracting (called matrixing)
to obtain the separate L and R channels. Then two audio channels (L and
R) and two speakers could be used to obtain true stereo reproduction.
A stereo generator module or system must be used to process the incoming
audio channels into a form suitable for transmission by a standard FM
transmitter on a standard FM channel 200 kHz wide, with 75-kHz peak deviation.
The usual preemphasis and deviation limiting must be provided for, and
the frequency response of the audio should not be degraded from standard
mono transmissions. Because avail able deviation is limited by FCC regulations
to 75 kHz, the L and R channel total audio must not exceed that for an
equivalent mono channel. Therefore, each channel can supply half of the
peak deviation. In addition, a pilot signal is needed for demodulating
the audio subcarrier, and some provision must be made for the use of
auxiliary subcarriers, such as Secondary (or Subsidiary) Communications
Authorization (SCA) transmissions on 67 or 92 kHz. Therefore, the audio
channels must be limited in practice to contributing about 80 percent
of the full deviation so there is room left for the pilot and SCA subcarriers.
This level difference is hardly notice able in practice on the received
signal. The circuitry needed for all of this processing, although involving
several components, is simple and straightforward, involving only low
frequencies, and is easily implemented with a few integrated circuits
(ICs) and transistors.
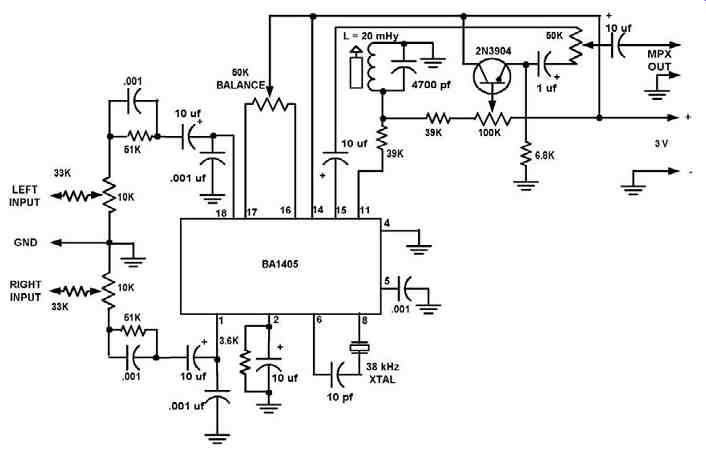
FIG. 1 Stereo Generator Using a BA1405
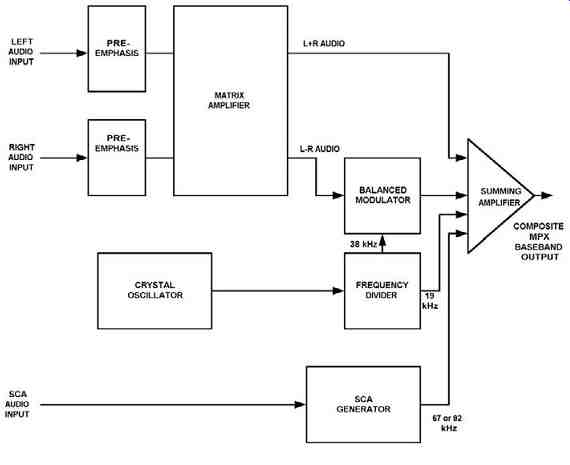
FIG. 2 Block Diagram of an FM Multiplex Generator
Indeed, there once existed an IC that did the whole job (BA1404 or BA1405),
but this IC has suffered the fate common to many ICs-manufacturer's discontinuance.
For some hobbyists and low-power transmitter manufacturers, this is
grim news. The folly in depending on specialized LSI chips that are not
second-sourced by at least two other manufacturers is well illustrated
here. Thousands of hobbyists' stereo FM transmitters both in kits and
individual projects were constructed around the BA1404/BA1405 ICs. Although
they may be available in surplus for a few years, their future is doubtful
because little mass-market demand for these chips exists.
They were fine for low-end uses, but real FM stereo demands performance
and signal-to-noise ratios well beyond the capabilities of these chips.
We show a circuit of a stereo generator using the BA1405 chip for illustrative
purposes only ( FIG. 1); however, it is not difficult to build a much
better stereo generator using discrete transistors and a few "classic" IC
devices that are widely available from several manufacturers, together
with a handful of resistors and capacitors, that will give "broadcast
quality" stereo sound to a low-power hobby transmitter. In addition,
all waveforms are accessible for study and learning how the stereo system
works.
The stereo generation process (see FIG. 2) is as follows: The two input
channels L and R are first fed to a preamplifier, where the audio is
amplified and pre-emphasized. This means that higher frequencies are
boosted relative to lower frequencies by a controlled amount. This is
done as a means of improving the signal to-noise (s/n) ratio of the received
audio. In the receiver, exactly the opposite is done (de-emphasis), which
reduces high-frequency receiver noise and produces an overall improved
s/n and therefore "cleaner" high frequencies. A simple RC lowpass
filter circuit is used for this task, and it may be either a distinct
RC circuit or incorporated into the frequency response of one of the
audio stages. In U.S. FM practice, a single 0 at 2.1 kHz, corresponding
to an RC circuit time constant of 75 microseconds, is used. Therefore,
below about 1000 Hz, no alteration is made to the audio.
At 1 kHz, a boost of around 1 dB occurs; at 2.1 kHz, a boost of 3 dB;
and at 4.2 kHz, a boost of 7 dB is used, and so on at 6 dB/octave until
15 kHz. The audio is then limited in amplitude by a limiter, generally
a diode peak clipper, to protect against over-modulating the transmitter.
This is done for both L and R channels. Pro gram input should be limited
in frequency to 15 kHz to avoid artifacts and aliasing effects and to
make the job of the lowpass filter easier in reducing these effects.
The stereo generation process assumes that no input frequencies above
15 kHz are present. Next, a lowpass filter is used to reduce audio components
above 15 kHz both input and those resulting from any clipping. In some
(European) systems, a preemphasis time constant of 50 microseconds, corresponding
to a 0 at 3.15 kHz, is used instead of 2.1 kHz.
Next, the processed audio channels are added and subtracted algebraically
in a matrix amplifier circuit. The (L+R) and (L-R) signals are now generated.
The (L+R) signal is the main component of the "baseband" signal
and is fed to a summing amplifier, where it is combined with other components
(to be discussed later). The output of this summing amplifier is fed
to the transmitter audio input.
The difference (L-R) signal must be separated in frequency from the
(L+R) signal to keep them separated while being transmitted on the same
channel. This is done by transforming the (L-R) signal into a double
sideband (DSB) suppressed carrier AM signal at a frequency high enough
so its lowest frequency components are far above the highest frequency
components in the main L+R signal. A balanced modulator circuit is used
for this operation (see FIG. 3). A carrier frequency of 38 kHz has been
standardized for this purpose, and a pilot signal of half this frequency
at 19 kHz is also generated. The pilot signal can be doubled at the receiver
and used to generate a local 38-kHz signal for demodulation of the subcarrier
into L-R audio, and it can be used to signify the presence of stereo
audio. The pilot carrier is also used by some receivers as a signal to
switch from mono to stereo and back to mono if the pilot at 19 kHz falls
below a minimum level, indicating insufficient signal for good stereo
reception.
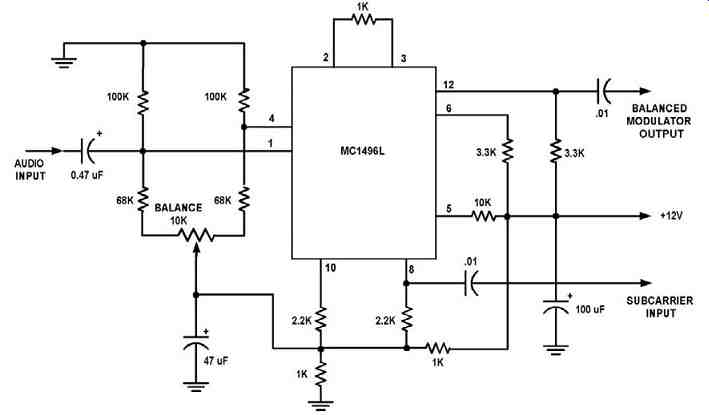
FIG. 3 Balanced Modulator Circuit Using an MC1496L
For TV stereo audio, 31.468 kHz and 15.734 kHz, which are two times
and one times the horizontal scan frequencies, can be used if the audio
response is limited to about 12 kHz or so. A DSB suppressed carrier signal
is used because if a standard AM signal were used, the carrier at 38
kHz would "hog" a large part of the allowable deviation of
75 kHz total permitted. This reaction would reduce the available deviation
for the sideband components, which really carry the desired audio information
for the FM, reducing the s/n ratio and contributing a filtering problem
in getting rid of the carrier component at 38 kHz, while keeping the
higher frequency audio components at 10-15 kHz relatively "clean." The
audio out of the balanced modulator has components from 23-53 kHz. This
corresponds to the sum and difference of the audio components at 0-15
kHz with the 38-kHz subcarrier (which is suppressed). This signal, along
with the 19-kHz pilot signal, is fed to the summing amplifier.
The pilot carrier and the subcarrier frequencies must be held to close
tolerances, preferably within 1 Hz or so. This requirement is not as
bad as it sounds, about 52 parts per million (ppm) for the pilot carrier
and 26 ppm for the subcarrier. Because the subcarrier is referenced to
the pilot, the 52 ppm tolerance at 19 kHz can easily be held with a crystal-controlled
oscillator. The 38-kHz signal can be generated and then divided by 2
to get the 19-kHz pilot. Although crystals for 38 kHz are available,
they can be delicate and expensive. A crystal in the 2-6 MHz range is
by contrast cheaper, more rugged, and easily available. Therefore, the
best approach is probably to use a crystal in the MHz range and to use
one of the CMOS divider chain chips available to divide the MHz frequency
by a power of 2 to get 38- and 19-kHz signals.
This approach is used in our circuits to be described in another section.
In these circuits, a 4.864-MHz crystal is used in an oscillator, and
its frequency is divided by 128 to get 38 kHz and then again by 2 to
get 19 kHz. Although the outputs from the divider are square waves, the
balanced modulator used to generate the subcarrier needs a square wave
anyway, and the pilot is inherently filtered by the bandwidth of the
audio system. Only two common digital CMOS ICs are used in this circuit,
and the cost is very low.
The output from the summing amplifier has the following spectral components:
1. The sum of the L and R channels (L+R) consisting of audio program
components from 0 (actually about 20 Hz) to 15 kHz. This is the part
received and used by mono FM receivers.
2. A pilot signal at 19 kHz of sufficient amplitude to produce around
10 percent of the total deviation permitted by the transmitter. This
signal is used for stereo detection and for regeneration of the 38-kHz
subcarrier at the receiver.
3. A DSB suppressed carrier signal having frequency components from
23-53 kHz, which carry the L-R signal needed by the stereo receiver.
4. Optionally, subcarriers at 57, 67, or 92 kHz used for data transmission,
or for SCA programming for use by private organizations. These subcarriers
generally have 7.5-kHz deviation and carry music or programming with
audio quality similar to AM broadcasting.
Therefore, a transmitter that handles FM stereo must have modulation
capability up to 100 kHz with reasonable flatness of frequency response.
Ideally, frequency response should be flat with constant time delay versus
frequency, which implies a linear phase response versus frequency. Distortion,
both harmonic and intermodulation, should be kept low to avoid crosstalk
and the production of unwanted spurious mixing products, which could
cause interference with audio programming. These components may show
up as noise, whistles, or loss of separation. Additionally, distortion
on the transmission path between transmitter and receiver must be avoided
as much as possible for best stereo reception. Multipath transmission
effects that occur at VHF frequencies can cause severe distortion and
ruin stereo reception. Antennas used with FM stereo transmitters and
receivers must have adequate bandwidth (rarely a problem) and enough
gain to ensure adequately received signal.
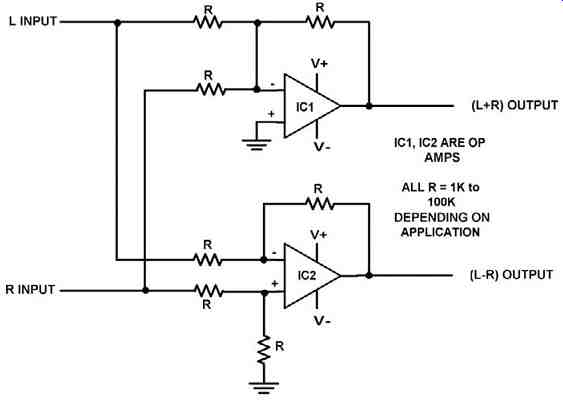
FIG. 4 Matrix Amplifier Used for Stereo Generation
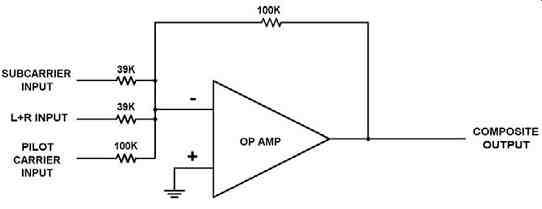
FIG. 5 Summing Amplifier Used for Stereo MPX Generation
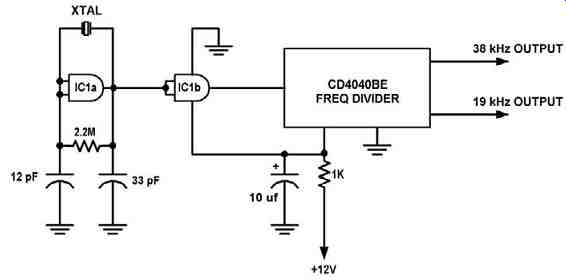
FIG. 6 Pilot and Subcarrier Generator FM Stereo Multiplex System
Some representative circuits are shown that can be used as building
blocks in a stereo generator system such as that just discussed. Figures
4, 5, and 6 are typical of these systems. Complete systems are
shown as parts of the MPX96 and MPX2000 discussions in later sections.
====
|