<<prev.
__6.3 Solving Interface Problems
__6.3.1 Ground Isolators
A device called a ground isolator solves the inherent common-impedance coupling
problem in unbalanced interfaces. Broadly defined, a ground isolator is a differential
responding device with high common-mode rejection. It is not a filter that
can selectively remove hum, buzz, or other noises when simply placed anywhere
in the signal path. To do its job, it must be installed where the noise coupling
would otherwise occur.
A transformer is a passive device that fits the definition of a ground isolator.
Transformers transfer a voltage from one circuit to another without any electrical
connections between the two circuits. It converts an ac signal voltage on its
primary winding into a fluctuating magnetic field that is then converted back
to an ac signal voltage on its secondary winding (discussed in detail in Section
11).
As shown in FIG. 43, when a transformer is inserted into an unbalanced signal
path, the connection between device grounds via the cable shield is broken.
This stops the noise current flow in the shield conductor that causes the
noise coupling, as discussed in Section 5.4. As discussed in Section 11, the
highest noise rejection is achieved with input-type transformers containing
Faraday shields. A transformer-based isolator for consumer audio signals using
such trans formers, the ISO-MAX model CI-2RR, is shown in FIG. 44. To avoid
bandwidth loss, such isolators must be located at the receive end of interconnections,
using minimum-length cables between isolator outputs and equipment inputs.
Conversely, isolators using output-type transformers, such as the ISO-MAX model
CO-2RR and most other commercial isolators, may be freely located but will
achieve significantly less noise rejection.
Ground isolators can also solve most of the problems associated with balanced
interfaces. The ISO-MAX® Pro model PI-2XX shown in FIG. 45 often improves CMRR
by 40 dB to 60 dB and provides excellent CMRR even if the signal source is
unbalanced. Because it also features DIP switches to reconfigure cable shield
ground connections, it can also solve pin 1 problems.
Because it uses input-type transformers, it attenuates RF interference such
as AM radio by over 20 dB. Again, to avoid bandwidth loss, it must be located
at the receive end of long cable runs, using minimum-length cables between
isolator outputs and equipment inputs. Other models are available for microphone
signals and other applications. The vast majority of commercial hum eliminators
and a few special-purpose ISO-MAX models use output-type transformers, which
may be freely located but offer significantly less CMRR improvement and have
essentially no RF attenuation.
Several manufacturers make active (i.e., powered) ground isolators using some
form of the simple differential amplifier shown in FIG. 31. Unfortunately,
these circuits are exquisitely sensitive to the impedance of the driving source.
FIG. 46 compares the measured 60 Hz (hum) rejection of a typical active isolator
to a transformer-based isolator. Over the typical range of consumer output
impedances, 100-ohm to 1 k-ohm, the trans former has about 80 dB more rejection!
Passive isolators based on input-type transformers have other advantages, too.
They require no power, they inherently suppress RF interference, and they're
immune to most overvoltages that can be sudden death to active circuitry.
__6.3.2 Multiple Grounding
When a system contains two or more grounded devices, such as the TV receiver
and the subwoofer power amplifier in our example home theater system, a wired
ground loop is formed as shown in FIG. 47.
As discussed in Section 5.3 and 5.4, noise current flowing in the shaded path
can couple noise into the signal as it flows in unbalanced cables or through
the equipment's internal the ground path. This system would likely exhibit
a loud hum regardless of the input selected or the setting of the volume control
because of noise current flow in the 20 ft cable. You might be tempted to break
this ground loop by lifting the safety ground at the subwoofer. Reread Section
4.2 and don't do it!
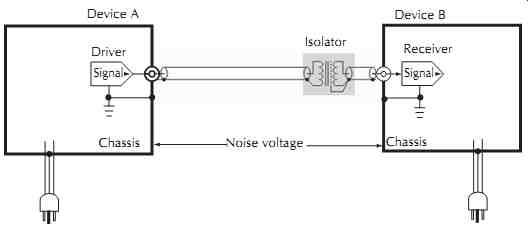
Figure 43. Ground isolator stops noise current in shield of unbalanced cable.
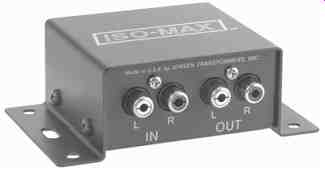
Figure 44. Stereo unbalanced audio isolator. Jensen Transformers,
Inc.
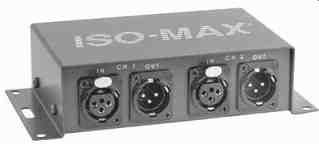
Figure 45. Stereo balanced audio isolator. Jensen Transformers,
Inc.
One safe solution is to break the ground loop by installing a ground isolator
in the audio path from preamp to subwoofer as shown in FIG. 48. This isolator
could also be installed in the path from TV receiver to preamp, but it is generally
best to isolate the longest lines since they are more prone to coupling than
shorter ones.
Another safe solution is to break the ground loop by installing a ground isolator
in the CATV signal path at the TV receiver as shown in FIG. 49. These RF isolators
generally should be installed where the cable connects to the local system,
usually at a VCR or TV input. If an RF isolator is used at the input to a splitter,
ground loops may still exist between systems served by the splitter outputs
since the splitter provides no ground isolation. Although it can be used with
a conventional TV or FM antenna, never install an RF isolator between the CATV
drop or antenna and its lightning ground connection (see Section 4.1). Isolators
will not pass dc operating power to the dish in DBS TV systems.
Since most unbalanced interfaces are made to consumer devices that have two-prong
ac plugs, isolating the signal interfaces may leave one or more pieces of equipment
with no ground reference whatsoever. This could allow the voltage between an
isolator's input and output to reach 50 Vac or more. While this isn't dangerous
(leakage current is limited in UL-listed devices), it would require unrealistically
high (CMRR over 140 dB) performance by the isolator to reject it! The problem
is solved by grounding any floating gear as shown in FIG. 50. This is best
done by replacing the two-prong ac plug with a three-prong type and adding
a wire (green preferred) wire connected between the safety ground pin of the
new ac plug and a chassis ground point.
A screw may be convenient as the chassis ground point. Use an ohmmeter to
check for continuity between the screw and the outer contact of an RCA connector,
which itself can be used if no other point is available.
Although, in the example above, an added ground at either the preamp or the
power amp would suffice, grounding the device with the highest leakage current-usually
those devices with the highest ac power consumption rating-will generally result
in the lowest noise floor.
__6.3.3 Unbalanced to Balanced and Vice Versa
The reader is referred to Section 11, Section 11.2.2, Audio Transformers,
for a more detailed discussion of these applications.
Beware of RCA to XLR adapters! FIG. 51 shows how using this adapter to connect
an unbalanced output to a balanced input reduces the interface to an unbalanced
one having absolutely no ground noise rejection! The potential noise reduction
benefit of the balanced input is completely lost.
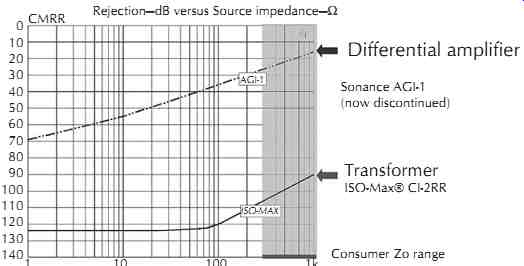
Figure 46. Measured hum rejection versus source impedance, active differential
amplifier versus input transformer isolator.
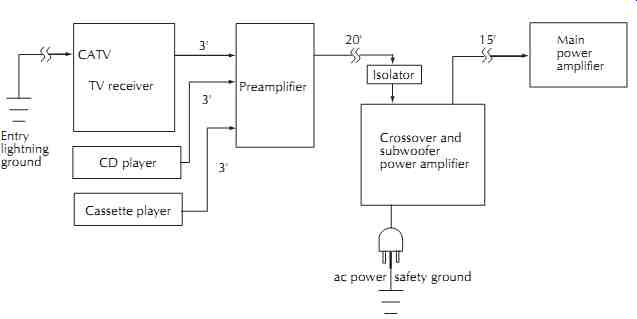
Figure 47. Using an audio ground isolator to break the loop.
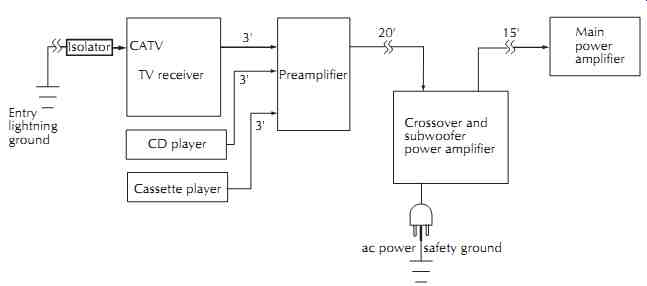
Figure 48. Using a CATV ground isolator to break the loop.
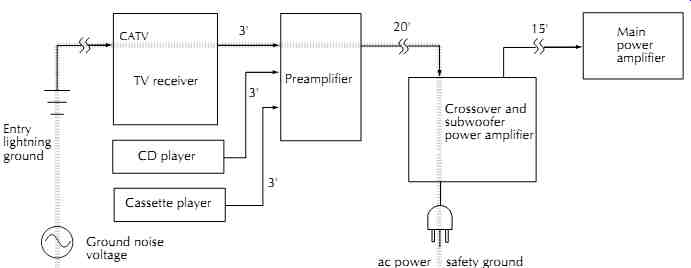
Figure 49. Loop created by two ground connections.
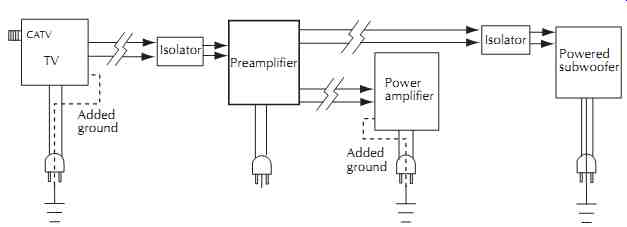
Figure 50. Grounding floating equipment when isolators are installed. From
Jensen AN004.
Proper wiring for this interface, shown in FIG. 52, results in at least 20
dB of noise rejection even if the balanced input is one of typically mediocre
performance. The key difference is that, by using shielded twisted pair cable,
the ground noise current flows in a separate conductor that is not part of
the signal path.
Driving an unbalanced input from a balanced output is not quite as straightforward.
Balanced equipment outputs use a wide variety of circuits. Some, such as the
one in FIG. 53, might be damaged when one output is grounded. Others, including
most popular servo-balanced output stages, can become unstable unless the output
is directly grounded at the driver end, which reduces the interface to an unbalanced
one with no noise rejection.
Unless a balanced output already utilizes a built-in trans former, using an
external ground isolator such as the one shown in FIG. 53 is the only method
that will simultaneously avoid weird or damaging behavior and minimize ground
noise when used with virtually any output stage.
This approach is used in the ISO-MAX® Pro model PC-2XR pro-to-consumer interface.
__6.3.4 RF Interference
As mentioned earlier, immunity to RF interference or RFI is part of good equipment
design. Testing for RFI susceptibility is now mandated in Europe. Unfortunately,
much of the equipment available today may still have very poor immunity. Under
unfavorable conditions, external measures may be needed to achieve adequate
immunity.
For RF interference over about 20 MHz, ferrite clamshell cores shown in FIG.
54, which are easily installed over the outside of a cable, can be very effective.
Some typical products are Fair-Rite #0431164281 and Steward #28A0640-0A. In
most cases, they work best when placed on the cable at or near the receive
end. Often they are more effective if the cable is looped through the core
several times.

Figure 51. Incorrect connection of an unbalanced output to a balanced input.

Figure 52. Correct connection of an unbalanced output to a balanced input.
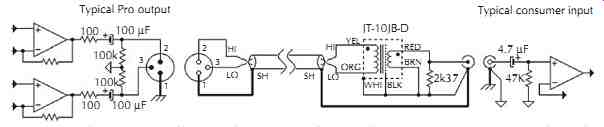
Figure 53. Bullet-proof connection of balanced output to unbalanced input.
Use the shortest possible cable on the RCA side to avoid common-impedance coupling.
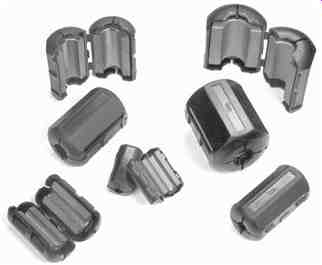
Figure 54. Ferrite cores.
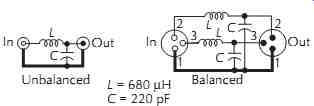
Figure 55. RF interference filters for audio lines.
If this is inadequate, or the frequency is lower (such as AM radio) you may
have to add a low-pass--i.e., high-frequency reject-RFI filter on the signal
line.
FIG. 55 shows sample 50 kHz cutoff, 12 dB per octave low-pass RFI filters
for unbalanced or balanced audio applications. For best performance and audio
quality, use NP0 (also called C0G)-type ceramic capacitors keeping leads as
short as possible, under ¼ inch preferred. For stubborn AM radio interference,
it may help to increase the value of C up to about 1000 pF maximum. The 680
µH inductors are small ferrite core types such as J.W. Miller 78F681J or Mouser
434-22-681. If the only interference is above about 50 MHz, a ferrite bead
may be used for L. For the balanced filter, inductors and capacitors should
be ±5% tolerance parts or better to maintain impedance balance.
The balanced filter can be used for low-level micro phone lines, but miniature
toroidal inductors are recommended to minimize potential hum pickup from stray
magnetic fields. These filters, too, are generally most effective at the receive
end of the cable.
When possible, the best way to deal with RF inter ference is to control it
at its source. FIG. 56 is a schematic of a simple interference filter for solid-state
120 Vac light dimmers rated up to 600 W. It begins attenuating at about 50
kHz and is quite effective at suppressing AM radio interference. It must be
installed within a few inches of the dimmer and, unfortunately, the components
are large enough that it usually requires an extra-deep or a double knock-out
box to accommodate both dimmer and filter. Parts cost is under $10.
A speaker cable can also become an antenna. In a strong RF field, enough voltage
can be delivered to the semiconductors in the power amplifier that they become
a detector and interference can be heard in the speaker even though the amplifier
may be unpowered! More commonly, this problem occurs when the amplifier is
powered and RF enters its feedback loop. In either case, the solution depends
on the frequency of the interference. Ferrite cores on the cable near the amplifier
may
help. In stubborn cases, a 0.1 µF or a 0.22 µF capacitor directly across the
output terminals of the amplifier may also be required.
__6.3.5 Signal Quality
Audio transformer design involves a set of complex tradeoffs. The vast majority
of available audio trans formers, even when used as directed, fall short of
professional performance levels. As Cal Perkins once wrote, "With transformers,
you get what you pay for.
Cheap transformers create a host of interface problems, most of which are
clearly audible." The frequency response of a high-quality audio trans
former is typically ruler flat, ±0.1 dB from 20 Hz to 20 kHz and -3 dB at 0.5
Hz and 100 kHz. The extended low-frequency response is necessary to achieve
low phase distortion. The high-frequency response is tailored to fall gradually,
following a Bessel function.
This, by definition, eliminates overshoot on square waves and high-frequency
response peaking. Dramatic improvements in sonic clarity due to the Bessel
filter action are often reported by Jensen customers who add transformers at
power amplifier inputs. On the other hand, cheap transformers often have huge
ultrasonic peaks in their response that are known to excite particularly ugly
intermodulation distortions in even the finest downstream power amplifiers.
Accurate time domain performance, sometimes called transient response, requires
low phase distortion to preserve musical timbre and maintain accurate stereo
imaging. Phase distortion not only alters sonic quality, it can also have serious
system head room effects. Even though it may have a flat frequency response,
a device having high phase distortion can increase peak signal amplitudes up
to 15 dB. Phase distortion should never be confused with phase shift. Linear
phase shift with frequency is simply a benign time delay: only deviations from
linear phase or DLP create true phase distortion. This DLP in a high-quality
audio transformer is typically under 2° across the entire audio spectrum.
Harmonic and intermodulation distortion in audio transformers is unusually
benign in character and cannot fairly be compared to electronic distortion.
By their nature, transformers produce the most distortion when driven at high
levels at very low frequencies, where the major distortion product is third
harmonic. Transformer distortion mechanisms are frequency selective in a way
that amplifiers, for example, are not. Electronic nonlinearities tend to produce
harmonic distortions that are constant with frequency while high-quality transformer
harmonic distortions drop to well under 0.001% at frequencies over a few hundred
Hz. Transformers also tend to have remarkably low intermodulation distortion
or IMD, to which the ear is particularly sensitive.
Compared to an amplifier of comparable low-frequency harmonic distortion,
a transformer typically has only a tenth the IMD. While cheap audio transformers
use steel cores producing 1% low-frequency harmonic distortion at any signal
level, high-quality transformers use cores of special nickel-iron-molybdenum
alloys for vanishingly low distortion.
Of course, noise rejection or CMRR is often the most important property of
a ground isolator. As discussed in Section 6.3.1 and Section 11, a trans former
requires an internal Faraday shield (not a magnetic or case shield) to maximize
CMRR. Most commercial isolators or hum eliminators consist of tiny imported
telephone-grade transformers that do not contain such a shield. Beware of products
with vague or nonexistent specs! For example, distortion described as under
0.1% is meaningless because frequency, signal level, and source impedance are
not specified. The most common problems with inexpensive isolators are marginal
noise reduction, loss of deep bass, bass distortion, and poor transient response.
Of course, ad copy and specifications of these transformers will put on their
best face, withholding the ugly truth! However, isolators using well-designed
and properly applied audio trans formers qualify as true high-fidelity devices.
They are passive, stable, reliable, and require neither trimming, tweaking,
nor excuses.
__6.3.6 Tips for Balanced Interfaces
Be sure all balanced line pairs are twisted. Twisting is what makes a balanced
line immune to interference from magnetic fields. This is especially important
in low-level microphone cabling. Wiring at terminal or punch-down blocks and
XLR connectors is vulnerable because the twisting is opened up, effectively
creating a magnetic pickup loop. In very hostile environments, consider starquad
cable because it has less susceptibility to magnetic fields. Magnetic coupling
is also reduced by separation distance, cables crossing at right angles rather
than running parallel, and shielding with magnetic material such as steel EMT
conduit.
Pay attention to cable shield grounding. As discussed in Section 5.3, the
shield must be grounded at the driven end, it may be grounded at both ends,
but never grounded only at the receive end. As a standard practice, grounding
at both ends is recommended for two reasons:
1. If the device input has marginal RF suppression, grounding the shield at
the input will usually reduce problems,
2. It doesn't require the use of a specially wired cable that might find its
way into another system and cause unexpected problems. If special cables are
made-to deal with a pin 1 problem, for example-be sure they are clearly marked.
Don't terminate to reduce noise. Nearly every practical audio system should
use unterminated audio circuits. This is standard professional audio practice
worldwide. While a 600-ohm termination resistor at an input may reduce noise
by up to 6 dB or more, depending on the driver output impedance, it will also
reduce the signal by the same amount, so nothing is gained. If noise is caused
by RF interference, installation of a suitably small capacitor at the input
may be much more appropriate.
Use ground isolators to improve noise rejection. As discussed in Section 4.1,
common balanced input circuits have generally unpredictable noise rejection
in real-world systems. Actual in-system CMRR can be as little as 30 dB when
using balanced sources and as little as 10 dB when using unbalanced sources.
A quality transformer-based ground isolator can increase the CMRR of even the
most mediocre balanced input to over 100 dB.
Beware of the pin 1 problem. As much as 50% of commercial equipment, some
from respected manufacturers, has this designed-in problem. If disconnecting
the shield at an input or output reduces a hum problem, the device at one or
the other end of that cable may be the culprit. See Section 5.3 for test methods.
Loose connector-mounting hardware is a major cause of pin 1 problems. Never
overlook the obvious!
__6.3.7 Tips for Unbalanced Interfaces
Keep cables as short as possible. Longer cables in crease the coupling impedance.
Serious noise coupling is nearly certain with 50 ft or 100 ft cables. Even
much shorter cables can produce severe problems if there are multiple grounds.
And never coil excess cable length.
Use cables with heavy gauge shields. Cables with shields of foil and thin
drain wires increase the common-impedance coupling. Use cables with heavy braided
copper shields, especially for long cables. See Section 7.4 for a recommended
high-performance cable. The only property of cable that has any significant
effect on audio-frequency noise coupling is shield resistance, which can be
measured with an ordinary ohmmeter.
Bundle signal cables. All signal cables between any two boxes should be bundled.
For example, if the L and R cables of a stereo pair are separated, nearby ac
magnetic fields will induce a current in the loop formed by the two shields,
causing hum in both signals. Like wise, all ac power cords should be bundled.
This will tend to average and cancel the magnetic and electro static fields
they radiate. In general, keeping signal bundles and power bundles separated
will reduce coupling.
Maintain good connections. Connectors left undisturbed for long periods can
oxidize and develop high contact resistance. Hum or other interference that
changes when the connector is wiggled indicates a poor contact. Use a good
commercial contact fluid and/or gold-plated connectors to help prevent such
problems.
Don't add unnecessary grounds! Additional grounding almost always increases
circulating noise currents rather than reducing them. As emphasized earlier,
never disconnect or defeat the purpose of safety or lightning ground connections
to solve a noise problem-the practice is both illegal and very dangerous!
Use ground isolators at problem interfaces. Transformer-based isolators magnetically
couple the signal while completely breaking the noise current path through
the cable and connectors. This eliminates common-impedance coupling and can
improve immunity to RF interference as well.
Predict and solve problems before an installation. For systems that consist
mostly of devices with two-prong power cords, some very simple multimeter measurements
on each system device and cable makes it possible to actually predict hum levels
and identify the problem interfaces before a system is installed.
__7 Alternative Treatments and Pseudoscience
The audio industry, especially the high-end segment, abounds with misinformation
and myth. Science, evidence, and common sense are often discarded in favor
of mysticism, marketing hype, and huge profits.
Just remember that the laws of physics have not changed! See FIG. 57.
__7.1 Grounding from Technical to Bizarre
In most commercial buildings, the ac outlets on any branch circuit are saddle
grounded or SG-types mounted in metallic J-boxes. Since SG outlets connect
their safety ground terminals to the J-box, the safety ground network may now
be in electrical contact with plumbing, air ducts, or structural building steel.
This allows coupling of noisy currents from other loads- which might include
air conditioning, elevators, and other devices-into the ground used by the
sound system. In a scheme called technical or isolated grounding, safety grounding
is not provided by the J-box and conduit but by a separate insulated green
wire that must be routed back to the electrical panel alongside the white and
black circuit conductors to keep inductance low. The technique uses special
insulated ground or IG outlet-marked with a green triangle and some times orange
in color-which intentionally insulates the green safety ground terminal from
the outlet mounting yoke or saddle. The intent of the scheme is to isolate
safety ground from conduit. Noise reduction is some times further improved
by wiring each outlet as a home run back to the electrical panel or subpanel,
making each outlet essentially a separate branch circuit. This technique is
covered by NEC Article 250-74 and its exceptions. In many cases, simply adding
a new branch circuit can be just as effective yet far less expensive than implementing
a technical ground system.
Many people, believing that the earth simply absorbs noise, have a strong
urge to install multiple earth ground rods to fix noise. This is desperation-mode
thinking.
Code allows extra ground rods, but only if they are bonded to an existing
properly implemented safety ground system. Code does not allow them to be used
as a substitute soil resistance is simply too high and unstable to be relied
on to divert fault currents.
Equipment grounding via the standard power cord safety ground is logical,
easy to implement, and safe.
It's highly recommended for all systems and is the only practical method for
portable or often reconfigured systems.
__7.2 Power-Line Isolation, Filtering, and Balancing
Most sound systems use utility ac power. If it is disconnected, of course,
all hum and noise disappears.
This often leads to the odd conclusion that the noise is brought in with the
power and that the utility company or the building wiring is to blame. Devices
claiming to cleanse and purify ac power have great intuitive appeal and are
often applied without hesitation or much thought. A far more effective approach
is to locate, and safely eliminate, ground loops that cause coupling of noise
into the signal. This solves the real problem. In reality, when system designs
are correct, special power treatment is rarely necessary. Treating the power
line to rid it of noise is analogous to repaving all the highways to fix the
rough ride of a car. It's much more sensible to correct the cause of the coupling
by replacing the shock absorbers!
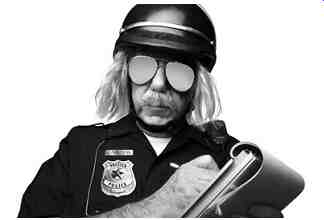
Figure 57. Officer Einstein of the Physics Police. Coil-Craft.
First, when any cord-connected line filter, conditioner, or isolation transformer
is used, Code requires that the device as well as its load still be connected
to safety ground as shown in FIG. 58. Cord-connected isolation transformers
cannot be treated as separately derived sources unless they are permanently
wired into the power distribution system per Code requirements.
Sometimes makers of isolation transformers have been known to recommend grounding
the shield and output to a separate ground rod. Not only does this violate
Code, but the long wire to the remote ground renders the shield ineffective
at high frequencies. It is a sobering fact that, while a device may control
interference with respect to its own ground reference, it may have little or
no effect at the equipment ground. Because all these cord-connected devices
divert additional 60 Hz and high-frequency noise currents into the safety ground
system, they often aggravate the very problem they claim to solve. External,
cord-connected filters, or those built into outlet strips, can serve to band-aid
badly designed equipment. As shown in FIG. 24 (Section 4.2), some equipment
is sensitive because common-mode power line disturbances, especially at high
frequencies, have essentially been invited in to invade the signal circuitry!
Second, the advertised noise attenuation figures for virtually all these power
line devices are obtained in a most unrealistic way. Measurements are made
with all equipment (generator, detector, and device under test) mounted to
a large metal ground plane. Although the resulting specs are impressive, they
simply don't apply to performance in real-world systems where ground connections
are made with mere wires or conduit.
However, these devices can be very effective when installed at the power service
entrance or a subpanel, where all system safety grounds are bonded to a common
reference point. For thorough, accurate information about separately derived
power distribution and its application to equipment racks, the author highly
recommends reference.
Balanced power, more properly symmetrical power, is another seductively appealing
concept shown in FIG. 59. If we assumed that each system box had neatly matched
parasitic capacitances from each leg of the power line to its chassis ground,
the resulting noise current flow into the safety ground system would be zero,
the inter-chassis voltage would be zero, and the resulting system noise due
to these currents would simply disappear! For example, if C1 and C2 had equal
capacitance and the ac voltages across them were equal magnitude but opposite
polarity, the net leakage current would indeed be zero. However, for the overwhelming
majority of equipment, these capacitances are not equal or even close. In many
cases, one is several times as large as the other-it's just a reality of power
trans former construction. Even if the equipment involved has two-prong ac
power connections, actual noise reduction will likely be less than 10 dB and
rarely exceed 15 dB.
And it's unlikely that equipment manufacturers will ever pay the premium to
match transformer parasitic capacitances or use precision capacitors in power
line EMI filters. If the equipment involved has three-prong (grounding) ac
power connections, the leakage current reduction, if any, provided by symmetrical
power will pale by comparison to the magnetically induced voltage differences
described in Section 3.4. In fact, many of the benefits attributed to symmetrical
power may result from simply plugging all system equipment into the same outlet
strip or dedicated branch circuit-which is always a good idea.
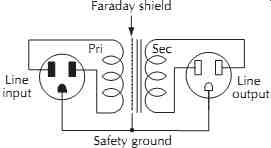
Figure 58. Power isolation transformer. Faraday shield; Safety ground; Line
input Pri Sec Line output
A GFCI (ground-fault circuit interrupter) works by sensing the difference
in current between the hot and neutral connections at an outlet. This difference
represents current from the hot conductor that is not returning via neutral.
The worst-case scenario assumes that the missing current is flowing through
a person.
When the difference current reaches 4-7 mA- producing a very unpleasant but
non-life-threatening shock-an internal circuit breaker removes power in a fraction
of a second. Some power conditioners feature a ground lift switch, touted to
eliminate ground loop problems, at their outputs. The National Electrical Code
requires that all balanced power units have GFCI-protected outputs (among other
restrictions on the use of balanced power). Although safe, ground lifting makes
a GFCI-protected circuit prone to nuisance trips.
For example, consider the system hook-up shown in FIG. 60.

Figure 59. Balanced power hopes to cancel ground currents.
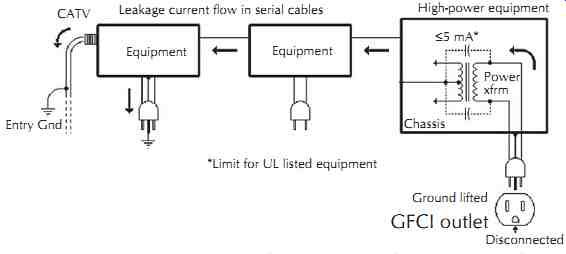
Figure 60. Common scenario to produce nuisance trips of GFCI in power conditioner.
For equipment having a grounding (three-conductor) power cord, UL listing
requires that its leakage current be no more than 5 mA. Normally, this current
would flow through the safety ground path back to neutral and would not trip
a GFCI that has an intact safety ground connection. However, if the safety
ground is lifted and the equipment is connected to other system equipment via
signal cables, the leakage current will flow in these cables to reach ground,
and ultimately neutral. Because the current is not returning via the equipment's
own power cord, the GFCI considers it hazardous and may trip, since 5 mA is
within its trip range. If multiple pieces of equipment are plugged into a single
GFCI-protected circuit, the cumulative leakage currents can easily become high
enough to trip the GFCI. This problem severely limits the ability of the GFCI/
ground-lift combo to solve ground loop problems--even when balanced power partially
cancels leakage currents.
__7.3 Surge Protection
Haphazard placement of common surge protectors can actually result in damage
to interface hardware if the devices are powered from different branch circuits.
As shown in FIG. 61, very high voltages can occur should there be an actual
surge. The example shows a common protective device using three metal-oxide
varistors, usually called MOVs, which limit voltage to about 600 Vpeak under
very high-current surge conditions.
For protection against lightning-induced power line surges, this author strongly
recommends that MOV protective devices, if used at all, be installed only at
the main service entry. At subpanels or on branch circuits to protect individual
groups of equipment, use series-mode suppressors, such as those by Surge-X,
that do not dump surge energy into the safety ground system, creating noise
and dangerous voltage differences.
__7.4 Exotic Audio Cables
In the broadest general meaning of the word, every cable is a transmission
line. However, the behavior of audio cables less than a few thousand feet long
can be fully and rigorously described without transmission line theory. But
this theory is often used as a starting point for pseudotechnical arguments
that defy all known laws of physics and culminate in outrageous performance
claims for audio cables. By some estimates, these specialty cables are now
about a $200 million per year business.
Beware of cable mysticism! There is nothing unexplainable about audible differences
among cables. For example, it is well known that the physical design of an
unbalanced cable affects common-impedance coupling at ultrasonic and radio
frequencies. Even very low levels of this interference can cause audible spectral
contamination in downstream amplifiers.
Of course, the real solution is to prevent common-impedance coupling in the
first place with a ground isolator, instead of agonizing over which exotic
cable makes the most pleasing subtle improvement. Expensive and exotic cables,
even if double or triple shielded, made of 100% pure unobtainium, and hand
braided by Peruvian virgins, will have NO significant effect on hum and buzz
problems! As discussed in Section 5.4, shielding is usually a trivial issue
compared to common-impedance coupling in unbalanced interfaces.
It's interesting to note that some designer cables selling for $500/meter
pair have no overall shield at all-ground and signal wires are simply woven
together.
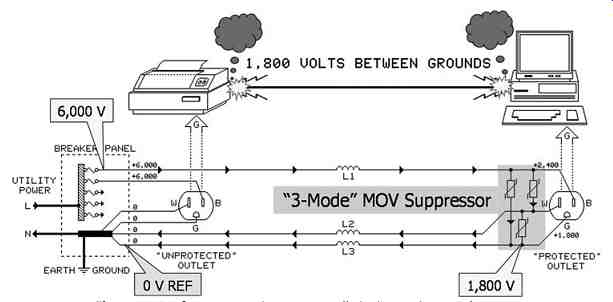
Figure 61. Surge protection can actually invite equipment damage.
Some exotic audio cables have very high capacitance and can seriously degrade
high-frequency response, especially if cables are long and/or a consumer device
drives it. For demanding high-performance applications, consider a low-capacitance,
low-shield-resistance cable such as Belden #8241F. Its 17 pF/ft capacitance
allows driving a 200 ft run from a typical 1 k: consumer output while maintaining
a -3 dB bandwidth of 50 kHz.
And its low 2.6 m:/ft shield resistance, equivalent to #14 gauge wire, minimizes
common-impedance coupling. It's also quite flexible and available in many colors.
Suggested Reading:
Henry Ott, Noise Reduction Techniques in Electronic Systems, Second Edition,
John Wiley & Sons, 1988.
Ralph Morrison and Warren Lewis, Grounding and Shielding in Facilities, John
Wiley & Sons, 1990.
Ralph Morrison, Grounding and Shielding Techniques in Instrumentation, Third
Edition, John Wiley & Sons, 1986.
part 1 | part
2 | part 3 | part 4 |